Flash and JavaScript are required for this feature.
Download the video from iTunes U or the Internet Archive.
Description: In this lecture, Prof. Liu further explains two sides of the AdS / CFT duality, including a brief overview of string theory and gravity in AdS spacetime.
Instructor: Hong Liu
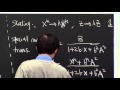
Lecture 17: More on AdS / C...
The following content is provided under a Creative Commons license. Your support will help MIT OpenCourseWare continue to offer high-quality educational resources for free. To make a donation or to view additional materials from hundreds of MIT courses, visit MIT OpenCourseWare at ocw.mit.edu.
HONG LIU: So let me first just remind you briefly what we did in last lecture--
AUDIENCE: [INAUDIBLE].
HONG LIU: --which was ages ago.
[LAUGHTER]
So we, say, quote unquote, derived the AdS duality. And then we also described a little bit to the geometry of the Anti-de Sitter spacetime. So there are two things I want you to remember about Anti-de Sitter spacetime. You said, there's two ways to think about it. One is so-called the Poincare patch, is that, say, we have a coordinate z or R. It depends on your choice. So for each constant-- z is equal to 0. Add to the boundary of AdS. So AdS have a boundary, symmetric, yeah, so R squared.
So z goes from 0 to infinity. And at z equal to 0, the overall [? prefect ?] blows up. So the spacetime become bigger and bigger. So this is what we will normally call the AdS boundary. And then when z become bigger, then you go to the interior.
And then at each consonant z the spacetime is [INAUDIBLE] Minkowski space, say, our D dimension in Minkowski space. So this give you an AdS d plus 1. OK, so this gave you an AdS d plus 1. And so of each z [INAUDIBLE], it's an AdS space. So this is so-called the Poincare patch.
And another way to think about AdS is so-called the global AdS. In the global AdS, AdS is just essentially a [? sort ?] in the cylinder. And it is a time direction, which we call tau. And then there's a radial direction we call rho. And then there's some angular direction. And then the boundary of the surface-- for example, you can write in this global case as 1 over, say R squared cosine squared rho, say, minus dt squared plus d rho squared plus sine squared rho [? d ?] omega squared d minus 1 squared. OK? And this rho, and the angular direction is this omega d minus 1, so d tau squared. OK?
And then when [? rho ?] goes to pi over 2, then the, again, the overall [? prefactor ?] blows up. So at the rho power over 2, so this is the boundary. So the boundary here is S3 times R. So SD minus 1 times the time. So it's just really a cylinder. So here, the boundary is the R1 d minus 1, just the Minkowski spacetime, OK?
So this is two way I want you to think about the Anti-de Sitter spacetime. And also, yeah-- so before we continue, do you have any questions? Yes?
AUDIENCE: Just a short one. So you draw a cylinder, But the spatial part of that metric is not precisely a cylinder.
HONG LIU: Hm?
AUDIENCE: I mean, the spatial part of the metric is not precisely [INAUDIBLE].
HONG LIU: You mean the boundary?
AUDIENCE: No, I mean the sine squared rho term. That's a cylinder.
HONG LIU: Oh, I'm just saying the topology. I'm saying the topology is [? a sorted ?] cylinder. Yeah, the topology is [? a sorted ?] cylinder, yeah. Any other questions? OK, good. So also, let me remind you this original definition of the Anti-de Sitter spacetime.
So Anti-de Sitter spacetime can also be defined as a hyperboloid. Hyperboloid in the d plus 2 dimensional Minkowski spacetime. OK, so this is the AdS d plus 1 can also be defined as hyperboloids in the d plus 2 dimensional Minkowski spacetime. It's 2 time. So it's a 2,d signature with 2 time. OK?
So now, let's talk a little bit more about the-- the last time we talked about geometry of AdS. Now, we talk about little bit the symmetries of AdS. So let's just further that discussion, so d plus 1 dimension of AdS, Anti-de Sitter spacetime.
So I assume all of you are familiar of is the concept called isometry. If not, it's very easy to understand. So isometry refers to the class of coordinate transformations, which leaves the metric invariant. OK? And it's, say, if it's a Minkowski spacetime, then the isometry will the Lorentz transformation plus the translations, OK? So that's the isometries.
And so for this AdS, by definition, the isometry would be SO(d,2), because this is hyperbole for AdS, because the AdS is defined as a hyperboloid in this d plus 2 dimensional Minkowski spacetime with signature (2,d). And this hyperboloid preserves the Lorentz transformation. So the AdS, the symmetry will be SO(d,2). Just the Lorentz symmetry of this embedded Minkowski spacetime. There's no translation because this equation breaks the translation. OK? Because this equation breaks the translation.
So yeah, so you can see immediately just from there. But what we will be useful later is to understand how this is refracted in the so-called Poincare patch. OK? So let's talk about how this symmetry is realized in the Poincare patch, which I will use these z coordinates, which I will use these z coordinates.
So first, they are translations, which we can translate, say, x mu to some constant. So by x mu, I always refer to using these Poincare coordinates. The x mu always refer to t and the vector x. OK? So this is the-- so first, obvious, there's a translation in the t and x direction, because that's independent here [? in the ?] x.
And then this also Lorentz transformation in the x direction, because of the dependence on the t and x is just the Minkowski metric, because each is [INAUDIBLE] that Minkowski metric. OK? So this has d generators. So this have 1/2 d times d minus 1, because this is the Lorentz transformation in the d dimension.
And then, also, there's scaling. So that metric, it's invariant under such a scaling. So just scale x mu and z together. And clearly, the metric does not change. So if you scale z and x together, then all the scaling cancels. No, of course, this does not change the metric. OK? So there's also scaling, so this have one generator.
So the last isometry in the-- it's called special conformal transformation. The last image of this space, which is called the special conformal transformation, it's a little bit more complicated. Let me just first write it down. Then I will try to give you some intuition about it.
So the [? free ?] parameter, so the parameter for this transformation of [? actor ?] b mu. OK? So I will introduce. So the transformation is the following. z go to z prime equal to-- and the x mu goes to x mu prime. OK? So b mu is just an arbitrary constant vector, just constant vector. And so b mu squared, so b squared is just your standard b mu b mu. Under the A square, it's defined to be z square plus x mu x mu. OK?
So you can check yourself, requires a little bit of effort. You plug this transformation into this expression. And then you can show that this is invariant. OK? So this have d parameters, because b. So the transformation parameters, they are the d parameters. So they are d parameters here. OK? Because of the b mu.
So this transformation can be understood in a slightly easier way, as follows. It's as you will also do this in your p set, is that you can check. So to understand this Special Conformal Transformation, so I called SCT. First, you can check the following is isometry, something we call inversion. It said if z goes to z divided by A, so A is this quantity.
Oh, A, I think I'm having some notation error, myself. So I should call it A, rather, A squared. Sorry. It doesn't matter. I can also call it A squared, but let me just call it A. I think that one would be [? A2. ?] Yeah, that is [? A2. ?] So, yeah, I could just call everything A squared. But to be consistent with my notation on the [? notes, ?] just let me call it A.
So you can also check that the following discrete transformation is also isometry, so when z equal to z divided by A, and x mu goes to x mu divided by A. OK? You can check this is isometry. So if you plug this into this metric, you find this [? leaves ?] the metric invariant.
And this thing is much easier to check. But as you will do in your p set, and you can check yourself, this discrete transformation actually is not part of the SO(d,2). It's, in fact, part of the O(d,2). So if you calculate its determinant, say the Jacobean, actually find the minus 1, rather than 1. OK? So this is not a proper Lorentz transformation. It's not a proper transformation, what we normally call the proper transformation.
But however, if you do this twice, the minus 1 times minus 1 equal to 1, then you get the proper transformation. But, of course, if you do this twice, you just go back to itself. You can check. This is inverse of itself. So this is like a [? z2 ?] transformation.
But you can do [? a slightly ?] [? trick ?]. Then you can show that this special conformal transformation is given by an inversion. You first do an inversion, and then you follow the [? bad ?] translation in b mu. And then invert it back. OK?
So even though you can check that I squared is equal to 1, well, now in the middle, you have added the translation in b mu, a constant. And this is a symmetry. And, of course, the whole thing will be a symmetry. But now, this is a proper transformation, because I have act I twice, OK? So you can now check this transformation is precisely-- if you do this, it's precisely just that transformation. OK?
So if you add all of them together, d will have d minus 1 d. Then you can show actually they form this symmetry group. They form this symmetry group. So altogether, you have d plus 1/2 d times d minus 1 plus 1 plus d. Then that give you 1/2 d. You can check yourself, like 1/2 d plus 1 and d plus 2, which is precisely the number generated for that one. OK? Yes?
AUDIENCE: So the total here that dimension, the d plus 1--
HONG LIU: Yeah.
AUDIENCE: Why like even translation, it only has a d dimensional invariant.
HONG LIU: No, because this depend on z, so the translation [? in ?] z is not invariant.
AUDIENCE: Oh, OK. Yeah, so that would be--
HONG LOU: Because this depend on z.
AUDIENCE: Yeah.
HONG LIU: So if you translate z, this is not the isometry [? under ?] the metric changes. So only the translation t and x is a symmetry. So that's why we only do the translation x mu.
AUDIENCE: I have some questions.
HONG LIU: Yes?
AUDIENCE: So conversion is isometry.
HONG LIU: Yeah.
AUDIENCE: Yes? OK, so but inversion, there's a [? release ?] not in the SO(d,2)?
HONG LIU: Yeah, right.
AUDIENCE: So that means the symmetry, isometry [? work ?] [? with ?] [INAUDIBLE] [? SO? ?]
HONG LIU: Yeah, yeah. It's the standard story. You have O. You can have an O symmetry. There's a discrete part, which is O/ When you're transformation, you're changing the Jacobean, whether your Jacobean is 1 or minus 1. You just actually [? exactly ?] the standards of the transformation in the Minkowski spacetime. In the Minkowski spacetime, you can also can see the [? site ?] transmissions.
AUDIENCE: OK.
HONG LIU: Yeah, just the same thing, because this is just the transformation in the Minkowski spacetime of this embedded space.
AUDIENCE: So in the [INAUDIBLE] metric, we also have a, like a-- determine [INAUDIBLE] transformation.
HONG LOU: Exactly the same as the standard Minkowski space.
AUDIENCE: OK.
AUDIENCE: But discrete part just like a [INAUDIBLE]?
HONG LIU: Oh, no, it depends. Yeah, in the old dimension, yeah, in the 3 plus 1 dimension, just the [? parity. ?] That's right. In the 2 plus 1 dimension, if we invert all directions, then it's not. Right? Yes?
AUDIENCE: Is there an easy way to see the special conformal symmetry of the system?
HONG LIU: Hm?
AUDIENCE: Is there an easy way to see a symmetry from the metric?
HONG LIU: Yeah, you just plug this in.
[LAUGHTER]
AUDIENCE: Well, I mean, how do you know that there is such symmetry, [? complicated ?] symmetries?
HONG LIU: No, just say another way to understand is to do this one. So this inversion is a much simpler transformation, which you can easily check [? its ?] isometry. And then this procedure will guarantee this is isometry. [? because ?] each step is isometry. Good? OK, so after talking about the-- so that concludes our very quick review about the geometry and properties of Anti-de Sitter spacetime.
AUDIENCE: But maybe one more quick question. So since you said the isometry [? bigger, ?] and so if you why you only answer on the [? SO? ?]
HONG LIU: It's the same thing. In Minkowski space, we can also separate the discrete transformations and consider the continuous part.
AUDIENCE: Oh.
HONG LIU: Yeah, so it's just exactly the same thing.
AUDIENCE: OK.
HONG LIU: Yeah. Certainly here, we can consider the inversion too. We just here, I'm considering the part, which connect to the [? identity ?].
AUDIENCE: OK.
HONG LIU: Any other question?
AUDIENCE: So is it true that in some dimensions the inversions [INAUDIBLE] isometry?
HONG LIU: I think-- no, I think actually more dimensions. This is not. In more dimension, this is [INAUDIBLE]. Yeah, it--
AUDIENCE: [INAUDIBLE].
HONG LIU: Inversion is always isometry in any dimension.
AUDIENCE: But it's just that the determinant [INAUDIBLE].
HONG LIU: No, it's always minus 1, any dimension.
AUDIENCE: Oh, OK.
HONG LIU: Yeah. Other questions? Good. So after talk about Anti-de Sitter spacetime, now we can talk a little bit about the string theory in Anti-de Sitter spacetime. OK? We can talk about string theory. Now, let's talk a little bit more about a string [INAUDIBLE] the distance [INAUDIBLE]. This discussion is very easy and essentially trivial because we know very little about this string theory in Anti-de Sitter spacetime.
[LAUGHTER]
And so there's not much to talk about. But there are a few general statements we can say. So there are a few general statements we can say. First is that both AdS 5-- so we have said that AdS 5 is the maximally symmetric space of negative curvature. And we all know from your really kindergarten years, that S5 is the maximal symmetric space of positive curvature. So this combined together is really a maximally symmetric space OK?
So this AdS 5 times S5, so AdS 5 times S5 is a homogeneous space, homogeneous spacetime, maximally symmetric homogeneous spacetime. So whatever string theory in this space, it's-- so the only scale in this space is the curvature, curvature radius, and which is the same everywhere. OK? It's homogeneous. It's the same everywhere.
So essentially, R, so this R will be just a single parameter to characterize the curvature of this spacetime, so single number, which characterize the curvature of the spacetime. So even the space is not homogeneous, and you have to specify which place, which curvature, et cetera. But this is the maximally symmetric space, homogeneous. So a single number captures the curvature everywhere.
So that means that in string theory on this spacetime, it's really just characterized by the following dimensionless parameter, so alpha prime divided by R squared. I mean, alpha prime is a dimensional parameter. But in the end, it's given by the following dimensionless parameter. And only for any physical process can only this dimensionless parameter goes in, not separate of them, because there's no other scales. i is the only scale, and alpha prime is the only [? other ?] scale. And so for any physics, the only dimension parameter can come in is this one.
And, of course, there's another dimensionless parameter is the gs, is what we call the string coupling. So essentially, the series is specified by these two parameters. OK? And depend on what you want, you can also construct the Newton constant. The Newton constant can be expressed in terms of gs and 1/2 prime. So in type IIB string, the relation, so we are talking about type IIB string, the relation between the Newton constant and the, say, alpha prime and the gs is given by the following.
So instead of a considering these two parameters, you can exchange gs by Newton constant. So you can alternatively consider, say, gn to the R to the power 8, or alpha prime to the R squared, or just characterized by these two dimensionless numbers. After your choice, sometimes this is more convenient, and sometimes, this is more convenient.
So if you talk about string theory, work with the string theory [? parse ?] [? integral, ?] et cetera, then this is more convenient. But if you think about the gravity, then the Newton constant appears naturally. And then this become more [? natural. ?] OK? So the fact of the theory is controlled by these two dimensionless parameters.
AUDIENCE: The R is a constant R?
HONG LIU: Yeah, same R. It's just the curvature radius over the spacetime. The whole spacetime, it's just controlled by the single R.
AUDIENCE: Yeah, yeah.
HONG LIU: Yeah, yeah, also, implicit in the discussion here, which is in the metric where I wrote last time, is that the curvature radius here is exactly the same as the curvature radius here. And so the single curvature radius characterizes the whole thing. OK? OK, good.
So this means that whatever quantum gravitational theory or string theory in this spacetime, you can consider q limit, based on this 2 dimensionless parameter. So first is so-called the classical gravity limits. So this is the limit, which the string coupling goes to 0.
So that means-- remember, the string coupling controls the [? loop ?] [? corrections ?] over the strings. And also, essentially, it's the fluctuation over the spacetime. And so the string coupling is 0. And also, the alpha prime divided by R squared goes to 0. OK?
So this alpha prime divided by R squared goes to 0. So this is can be considered as a point particle limit. Because alpha prime essentially characterize so the [? sides ?] of a string. And when the side of the string is much smaller than the curvature radius, and then essentially, it's a point particle limit. So this is the standard classical gravity limit. So in this region, we have classical gravity. And you cannot tell that those particles are strings. They're just like ordinary particles. OK?
So similarly, so g string [? to ?] [? 0 ?] can also be considered as the limit. The Newton constant goes to 0. It's the same thing. OK? It's same thing. And so in this region, [? you ?] get classical gravity. And more specifically, in our case, we get to have to be super gravity, which we briefly mentioned before.
So there's a lot of the region is called the classical string limit. In this case, the alpha prime divided by R squared can be arbitrary. Does not have to be small. So in this region, than the string [? G ?] [? fact ?] will be important. So you should be able-- then that means that the spacetime curvature radius is comparable to the string sides. Then in this case, you can no longer treat the string as a point particle.
But we still take g string goes to 0. So that means that the quantum fluctuation is small. The spacetime quantum fluctuation is small. So in this case, you still have a classical theory. But it's a classical string theory, rather than a classical gravity theory. OK? Any questions regarding this?
So there's another-- something else?
AUDIENCE: No.
HONG LIU: So there's one feature in this spacetime. So this is altogether 10 dimensional spacetime. There's one feature of this spacetime. It said S5 is a compact space with a finite volume. And AdS 5 is uncompact OK?
So normally, when you have a compact space, so it's convenient-- so S5 is compact. So it's actually convenient inside your situation to express whatever, say, 10 dimensional field in terms of-- yeah, it may be to not express, to expand, but in 10 dimensional fields, in terms of harmonics, [? inverse ?] 5. OK? Because there's a discrete set of harmonics on S5, you can always expand some 10 dimensional field on it.
So for example, if you have a scalar field, so supposing if you have a 10 dimensional scalar field, say we have x mu. We have z. So this is AdS5 part. And them let me call it omega 5, which is S5 part. Then you can always, say, so you can expand these scalar fields in terms of spherical harmonics on S5. OK? Then what you get is you get a tau field in AdS 5. And then this are spherical harmonics in S5. OK?
And in particular, when you do this expansion, than the higher harmonics, they will develop a mass, because of the curvature of the S5. So the lowest mode will be independent, say, of the coordinate on the S5, where the higher mode will depend on the coordinate of S5. And because of those dependence, because of the curvature of [? base ?] 5, they will develop a mass. So the lowest mode would be massless, then they will be followed by tau of massive modes, controlled by the [? size ?] of AdS 5. OK?
So you can do this for any field, any particular [? the ?] metric. So when you do this, essentially, only the massless graviton in 5 dimension will [? mediate ?] no [? range, ?] say, gravitation [? interactions ?] in AdS 5. So essentially, so the gravity, so at a long distance, the gravity is essentially 5 dimensional. OK? It's the same in 5 dimen-- because you can always reduce on S5.
So there's a slightly tricky thing here. But it's only-- it's that the size of S5, the curvature radius of S5 is actually the same as the curvature radius of AdS 5. So there's not really a hierarchy here in terms of the curvature radius. But there's still an important difference. These have infinite volume. These only have a finite volume. So these are compact. These are uncompact. OK?
AUDIENCE: But why the gravity [? doesn't-- ?]
HONG LIU: Hm?
AUDIENCE: But why the gravity doesn't say this string [? S5? ?]
HONG LIU: No, it-- [INAUDIBLE] [? this thing ?] radius-- this [? thing ?] S5. But just as I said, this is a compact part. You can always do a reduction. And then the higher graviton mode will develop a mass.
AUDIENCE: Oh.
HONG LIU: Yeah, we develop a mass, yeah, even though that mass is not very big. Yes?
AUDIENCE: So if we applied this to the real world, then what would estimate for R?
HONG LIU: Sorry?
AUDIENCE: What's the estimate of this radius, this curvature for if it's should be comparables are of the original worlds?
HONG LIU: [INAUDIBLE] the size of the universe.
AUDIENCE: But then doesn't it mean that we only should worry about the mass of this compact mode?
HONG LIU: Yeah.
AUDIENCE: Only on distances much longer than ours?
HONG LIU: Yeah, yeah. I mean, just saying, at very large distance, essentially is 5 dimensions. But it's still-- you can talk about the distance scale, et cetera. But here, R is the only scale. It doesn't matter how large is R. Everything matches against R. R provides your units.
AUDIENCE: Yes, but when we talk about distances shorter than R then--
HONG LIU: Yeah, if you talk about a distance shorter than R, it doesn't matter. It's like a 10 dimensional. It's like a 10 dimension [INAUDIBLE]. But AdS is uncompact, you can go as much distance as you want. I'm just saying, the story is slightly tricky because they have the same curvature radius. But I'm trying to describe it in the spirit. And also, mathematically, you can always, because S5 is uncompact, you can always do dimensional reduction on it. And this provides a convenient way to organize fields, in terms of 5 dimensional fields.
AUDIENCE: OK.
HONG LIU: OK. So one thing we will often use is, indeed, just consider the dimensional reduction of the gravity to 5 dimension. So if you have, say, say suppose this is Einstein-Hilbert action in 10d. So this is AdS 5 part. This is the S5 part. So you have some 10 dimensional, so curvature. So R is a [? rich ?] scalar in 10d. OK.
Now, suppose the metric, suppose this considered the lowest mode for the metric, which have low S5 dependence. And then you can just reduce this to a 5 dimensional theory. Gravity, so the volume of the S5 part, we are just [? factorize. ?] Then you have a 5 dimensional scalar. OK? And this b5 is just the volume of S5. OK? 5.
And now, we can absorb this b5 into downstairs, then define or you factor your 5 dimension Newton constant. 5 [INAUDIBLE] Newton constant, which we are call G5, which is defined to be GN divided by the volume of the 5 sphere. OK? And the volume of the 5 sphere is, essentially, just pi q. OK? And then, of course, there's R to the power [? of 5th. ?] So this is the quantity we will often use. OK?
So of the dimensional reduction, in the end, you get the action [? we will ?] have the form 14 pi G5, so 5 dimensional Newton constant, say, [? d5x ?], just AdS part. And then you have the gravity part of the action. And then you plus many, many matter fields. So this is, the essentially will be the structure of your action when you do the dimensional reduction. OK?
AUDIENCE: Shall we have matter fields in the S5?
HONG LIU: No, you reduce everything on S5. And once you have done that, then S5 will disappear.
AUDIENCE: But when we do, say, the R is [? always ?] a constant, so we can do the integral easily in S5. But if we don't have some mass [INAUDIBLE].
HONG LIU: Yeah, so no, it doesn't matter. So you can take-- this is a standard story for [INAUDIBLE] reduct-- a standard story. When you expand around in this, so this will go into here. Then the only dependence on the S5 part will depend on those things.
AUDIENCE: Uh-huh.
HONG LIU: Then you just can't integrate those things.
AUDIENCE: Oh.
HONG LIU: You can always integrate them.
AUDIENCE: Oh, OK.
HONG LIU: And they just give you some integrals, some numbers. It would just give you some numbers. And in the end, you can always write the action in terms of 5 dimensional action.
AUDIENCE: But that solution is a classical solution for the [INAUDIBLE] equation.
HONG LIU: No, no, no. This is not a classical solution. I'm just doing an expansion. It's just like doing a Fornier transform.
AUDIENCE: Oh, OK.
HONG LIU: This is expand in terms-- in some basis. No, have not done anything. This just a mathematical rewriting,
AUDIENCE: Mm-hm, OK.
HONG LIU: Yes?
AUDIENCE: But I think in terms of expression for G Newton into the affected-- don't we have that this G5 is like G squared alpha for the [INAUDIBLE] [? divided by ?] [? R to the 5th y ?] can put [? a use it ?] to this alpha R squared combination?
HONG LIU: Sorry--
AUDIENCE: Express G5 in terms of GS and alpha prime.
HONG LIU: Yeah?
AUDIENCE: And [INAUDIBLE]. Why don't it look like only combinations of R prime over R squared and GS?
HONG LIU: Sorry, I don't understand what you're asking. No, G5 is a dimensional parameter. 5 dimension Newton constant had dimensions 3. So whatever dimension here, some [? other ?] dimension there would be compensated by R5. But you always have 3 dimension left. So this is a dimension 3 number, yeah. Any other questions? Good.
OK, so then this concludes our discussion about string theory in AdS 5 times S5. As I said, there's not much to talk about it. And so now, let's look at the other object, which is the N equal to 4 super-Yang-Mills theory, which is the other side of the equation, of the duality equation. OK? So in this case, we have a lot to talk about it. We, in principle, have a lot to talk about it, but we won't have time. So we will also not talk much about it. So we are only mentioning a few essential things, OK?
So the field content, say, of a N equal to 4 super-Yang-Mills theory, which we already learned, which we already learned from the [INAUDIBLE] theory on the D3-brane is that you should have a gauge field. And then you have a 6 scalar field, because 1 into 6 transverse direction over the D3-brane. OK, you have 6 scalar fields.
And then when you include the super symmetric center, in the super string, then they actually also the massless of fermions. So this is 3 plus 1 dimension. OK? So this is 3 plus 1 dimension. So in 3 plus 1 dimension, you can represent the fermions conveniently by two components, for example, two component Weyl fermions.
They it turns out there are 4 such Weyl fermions. So A will go to 1 to 4. So alpha is a spinor indices. And A just neighbor different spinors. So altogether, you have four spinors. OK? And so this is, essentially, the field content of the N equal to 4 super-Yang-Mills theory. So we would not worry about the fermions. So but I'm just mention here for completeness.
So if you have a U(N) gauge theory, if you have a U(N) guage theory, say, for the N D3-brane, then all this, all this field is in the of the [INAUDIBLE] of U(N). In other words, each of them is an N by N matrix, is N by N Hermitian matrix. OK? So each of them can be represented by Hermitian matrices.
So altogether, yeah, yeah-- so let me not write it. Let me just say it in words. So altogether, you have 8 [INAUDIBLE]. You have 8 bosonic degrees of freedom. Because for the photon, you have 2. For photon, you have 2 [INAUDIBLE] degrees of freedom. And here, 6 scalar field, you have 8 [INAUDIBLE] degrees of freedom. And for the fermion, you can also count that the 4 Weyl spinors actually have 8 [INAUDIBLE] degrees of freedom.
So altogether, you have 8 N square. Each of them is an N by N matrix. So you have 8 N squared, say, [INAUDIBLE] bosonic degrees of freedom, and eight 8 N squared for mionic [INAUDIBLE] degrees of freedom. OK, for the--
AUDIENCE: Why would we have 8 fermions?
HONG LIU: Hm?
AUDIENCE: We have 8 fermions?
HONG LIU: No, we have 4 fermions, but each fermion have some spinor component.
AUDIENCE: Oh, OK, OK.
HONG LIU: Yeah.
AUDIENCE: Where did the number come from?
HONG LIU: Sorry?
AUDIENCE: Where did 4 fermions?
HONG LIU: That come from a calculation. This I cannot explain here. This, I can only quote this fact. It's just that if you work out the theory on the D3-brane, then [INAUDIBLE] there's 4 Weyl fermions, yeah, which we did not go through that, because we did not do super string. Yeah.
AUDIENCE: Should this come from the fact that it's where [INAUDIBLE]? Symmetry?
HONG LIU: Yeah, it's come from the super symmetry. Yeah, come form the string theory, super string theory. So in our discussion, we only discussed bosonic part. We never had time to discuss for mionic part, et cetera.
So let me now mention one important point, which I think is-- I hope is self-obvious, self-evident to you. It's that this U(N)-- actually, there's a U(1) which decouples, which U(1) decouples, just actually, let me just write down the Yang-Mills theory first. So let me write down the Yang-Mills theory first.
So you can write down the Yang-Mills theory, which we are actually wrote it down before. Let me only write down the bosonic part. So essentially, just given by Yang-Mills coupling trace. And this is a covariant derivative acting on the scalar, standard covariant derivatives. And the I and the j are all summed. OK? So I and the j are all summed.
So this is the Lagrangian, then plus the fermionic part. So this is the bosonic part, then plus the fermionic part. OK? Yes?
AUDIENCE: Are fermions coupled through 5 fields?
HONG LIU: Yeah, they're all coupled. They're all coupled.
AUDIENCE: How do they couple?
HONG LIU: Standard [INAUDIBLE] coupling. Yeah, so the key, so they couple through a particular coupling. The hosting, it just can show to have a single coupling. In principle, if you have so many fields, you can have many, many different possible couplings. You can have one coupling between them, one coupling between them, et cetera. You can have different components, but and self-couplings, et cetera. And under the key of N equal to 4 super-Yang-Mills theory is that every coupling, they equal up to some constant, up to some constant factors, which is precisely give you the supersymmetry.
Anyway, so I think it's obvious to you that you have a U(N) Yang-Mills theory that the U(1) part decouples. OK? OK, you unplug the couples. So U(N), you can always decompose it into SU(N) times U(1). Under the U(1) part, [INAUDIBLE]. So each A, each field is N by N matrix. And the U(1) part is the part which is proportional to the identity matrix. And the SU(N) part is the part which given by N by N trace this matrix. And so the U(1) part is proportional to the identity matrix.
So but you can immediately see from here, anything which is proportional to the identity matrix all the commentators will vanish. OK? So essentially, U(1) part is a free theory. So U(1) part is a free theory. Yeah, if this is not clear to you, you can easily convince yourself afterwards.
And this is also where-- this is also physically clear from the point of view, thinking that this is coming from the D-branes. Coming from the D-brane, you have, essentially, you have N D-brane together. And this Yang-Mills theory essentially describes the low energy dynamics of D-branes, brains, so how all these N D-branes interact with each other.
But no matter know how they [? co-communicate ?] [? interact ?] with each other, there's always a center of mass motion. Does not depend on their internal structure. And that central mass motion essentially is just this U(1). And the central mass motion always decouple. OK? So U(1) decouples. And you can do the check from here. A U(1) decouples. OK?
AUDIENCE: Yeah.
HONG LIU: Good. So now let me-- so the interacting part is just SU(N). OK? The interacting part is just SU(N). So now, let me say a few words regarding the properties of the theory. So first, it's that this theory has N equal to 4 supersymmetry. OK? So this is another important remark. This is just explain the name, just explains the name.
So in supersymmetric theories, so you have a transformation between boson and the fermion. OK? You have some-- the theory is invariant on the transformation between boson and fermion. So on the [? side of ?] transformation, the [? conserved ?] charge, so for [INAUDIBLE] transformation, then you have loss of charge. [? For ?] [? such ?] [? as ?] transformation and loss of charge can be described by a spinor. OK?
So normally, we say N equal to 1 supersymmetry if the super charge is given by a single Weyl spinor in 4 dimension. So N equal to 4 means that the total number of such [? conserved ?] charge is actually given by the 4 Weyl spinors in the 4 dimension. So it doesn't matter, so these have a number of supersymmetries. OK?
And also, if you know a little bit of supersymmetry, then this is actually the maximally allowed, the supersymmetry in 4 dimensions, for [INAUDIBLE] field theory. So this is actually maximum allowed supersymmetry. But this will not be important for us. OK?
But this will be. But the next point will be very important for us. It's that because of this theory is so symmetric, so G Yang-Mills coupling, its dimensionless, classically. OK? Because this is a 4 dimension, the Yang-Mills coupling is dimensionless, classically. And the same thing with our own QCD, our own QCD coupling this dimensionless, classically.
But then quantum mechanically, then the coupling actually changes with the scale, OK, because over the quantum corrections, et cetera. So generic coupling changes with scale. But N equal to 4 super-Yang-Mills theory is special. But this actually, this Yang-Mills coupling does not change with the scale, even at the quantum level. So the quantum level, this remains, so dimensionless coupling.
So using the technical term, for those of you who have studied the QC Yang-Mills theory, is that the beta function at the quantum mechanical level, the beta function for G Yang-Mills is actually 0, which means you can really treat this G Yang-Mills as a parameter, as a dimensionless parameter, even quantum mechanically. OK?
So this is a genuine parameter. In contrasting our QCD, there's no such parameter. OK? In QCD, we only have a scale. There's no parameter. Good? So any questions regarding these?
AUDIENCE: But you said you wouldn't know the coupling [INAUDIBLE] coupling because of [INAUDIBLE]?
HONG LIU: Yeah.
AUDIENCE: That means this is not [INAUDIBLE]?
HONG LIU: No, it's not. No, in the--
AUDIENCE: It's not [INAUDIBLE].
HONG LIU: In QCD, the Yang-Mills coupling is not the parameter. It's not the dimensionless parameter.
AUDIENCE: OK.
HONG LIU: It translates into a scale.
AUDIENCE: Yes.
HONG LIU: It translates into a scale.
AUDIENCE: So--
HONG LIU: It translates into a mass scale.
AUDIENCE: Yes, but can we find a massless parameter in [INAUDIBLE] like this?
HONG LIU: No, because the beta function is nonzero there.
AUDIENCE: Ah.
HONG LIU: Yeah, yeah because the beta function is nonzero there. OK? And also because of this, the beta function is 0. This theory is actually conformally invariant,
theory is actually conformally invariant. OK? So which we'll normally call, say, it's a conformal field theory, because it's a CFT.
So one way to understand this is because of the beta function is 0, essentially, the theory does not have a scale. So theory classical scale, invariant quantum mechanically remains scale invariant. And then you can show that there actually is a little bit more generous conformally invariant. So let me say a little bit about the-- are people familiar with the concept of the conformally invariant, or conformal?
AUDIENCE: No.
HONG LIU: No? OK. Yeah, so let me say a few words about the conformal. So conformally invariant means that the theory were under the conformal transformations. OK? So let me say a few words on the conformal transformations.
So conformal transformations are the following. Say, suppose your spacetime metric is given by some G mu mu. So the conformal transformations are those coordinate transformations. OK? You go from x to x prime. So that under such a transformation, your metric, the transformed metric is related to the original one only by an overall scale factor. OK?
So if lambda is equal to 1, then this is what we called earlier isometry, which leave the metric invariant, will be isometry. And if you leave the metric invariant after your overall scale factor, then this is called the conformal transformation. OK?
And for the Minkowski spacetime, for Minkowski spacetime, actually, I can just even use this thing here. Yeah, maybe let me-- yeah, let me erase here. So for Minkowski spacetime, say if the G mu mu is equal to [INAUDIBLE] mu mu-- suppose this is a d dimensional Minkowski spacetime-- OK? Then you can show-- you can just work how to solve this equation, work out all possible such transformations. OK? And you can show that conformal transformations are the following.
First, they are the standard isometry, which is the translation, because the translation does not give you lambda 1. And then you have a Lorentz transformation, which also give you lambda 1. OK? And they you can have a scaling. Of course, if you scale your coordinate with some factor, then, of course, the metric will only change by overall factor. So this is a translation. So this is s Lorentz symmetry. And this is scaling.
And then we also have something what we call a special conformal transformation. So this is for-- OK? And the b, again, is some constant parameter. OK? And also for the discrete transformation, also, you can have a discrete. Also you can have a discrete transformations inversion. You [? said ?] x prime mu equal to x mu divided by x squared. OK?
So these are all the transformations. In general dimension, which leave you metric invariant, leave the Minkowski metric invariant number up to overall factor. And the conformal invariant theory is a theory which [? involves ?] transformations. OK? And so obviously, a Yang-Mills theory are always invariant on the translation on the Lorentz transformation. But when the beta function is 0, the system does not have a scale. Then it's invariant on the scaling.
But typically, in general, 4 dimensional theory, any theory invariant on the scaling is also invariant on this special conformal transformations. So actually, the N equal to 4 super-Yang-Mills theory is actually conformal theory, OK, conformally invariant theory.
So now, as part of your p set, you can actually see that this conformal transformations in fact [? have ?] 1 to 1 correspondence with these isometries of the AdS. OK? And this we will see later. This is a part, important part of the relation between AdS. This is an important part of this duality relation. It's that the symmetry have to be the same. So you compare the two. You can see that, indeed, they 1 to 1 correspond to each other.
So the conformal transformation add together give you SO(d,2). OK? So this is the conformal group, so-called the conformal group in d dimension. Then you get to the [? SO(d,2). ?] OK?
And but in this theory, there's actually also global symmetry, because the N equal to 4 super-Yang-Mills theory come from the D3-brane, which is a transverse space, is the [? rotation of ?] invariant. So this actually our SO(6) symmetry rotates different phi i's. So actually, there's also global SO(6) symmetry, which rotates phi i, OK, and the fermions. OK?
And then when you include in the supersymmetry then you get a huge symmetry group. It's normally called a super conformal symmetry. Anyway, let me just write some notation down. It doesn't matter. So if you include the symmetry, the full symmetry group is what we normally call it. So this is a super group, so [? SU(2,2|4) ?] Doesn't matter.
So the bottom line is that the N equal to 4 super-Yang-Mills theory is the most symmetric 4 dimensional theory. OK? And almost-- no theory has more symmetries than N equal to 4 super-Yang-Mills theory. Any questions?
AUDIENCE: [INAUDIBLE].
HONG LIU: Yes?
AUDIENCE: So How do you know that the [? flows are ?] [INAUDIBLE]?
[? HONG LIU: Though ?] you can classify it by solving this equation. You can classify it by solving this equation.
AUDIENCE: But what's the q [INAUDIBLE]?
HONG LIU: No, this is just a notation.
AUDIENCE: A notation?
HONG LIU: Yeah. Any other questions? So I can say a few words about the conformal field theories. But it's getting a little bit late, and I want to talk about other things. So let me just say it in words. And then you can try to read it in other places. Because even if I just write a couple formulas here, it still won't change you very much.
[LAUGHTER]
I won't teach you too much. So important thing about this conformal field theory is that each operator, say each local operator-- yeah, you can classify local operators by how they transform, say, under these conformal symmetries. And then you can associate, say, a dimension to each operator, related to how they transform in these conformal transformations.
And then you can also show that the symmetry actually dictates the 2-point function and the 3-point function of such operators. And essentially, the structure of the 2-point function and 3-point function are completely fixed. But not higher point function, but only 2 and 3-point functions. Yeah, so that's essentially it.
Not much known, many things are known about conformal field theory in 1 plus 1 dimension. And but higher dimensions, yeah, other than what I said, not much. Not too much more is known, yeah. So any questions regarding this?
OK, so let me just summarize, then we can have a break. So we can summarize. So let me just summarize what we have done so far, and also, with an important refinement. So what we said is we started with two picture. One is D-brane with some open strings, and then some closed strings can interact with it. And then when we go to the low energy limit, then we get N equal to 4 super-Yang-Mills theory with, now, I say SU(N) plus a decoupled U(1), and then plus what we discussed last time, a decoupled the gravitons in the low energy limit. So let me just write E equal to 0 limit.
On the other side, we have this [? pure, ?] [? geometric ?] picture of the curved spacetime produced by those D-branes. So those curved spacetime at infinity behaves like just 10 dimensional Minkowski spacetime. And then when you go to the close to the brane, then the space deforms into the AdS 5 times S5. OK, form into AdS 5 times S 5.
Under the low energy limit, because 1 into-- we can see the string theory in the [? very ?] [? much ?] [? tongue ?] this [? throat. ?] OK? So the low energy limit is the string theory. The one here when you're take E equal to 0, we get string theory in AdS 5 times S5, then plus decoupled graviton.
Here, also, here, when we look at the geometry of the brane, essentially, we have fixed the location of the brane. So essentially, we have fixed to the center of mass motion. But in principle, you can allow, also, the brane to move anywhere. So here, essentially, there's also a decoupled center of mass motion. OK?
So now, let's get rid of this decoupled graviton. Let's get rid of this decoupled U(1), or decoupled center of mass motion. Then what we get is the fully interacting part. OK? So the fully interacting part is so small refinement than what we said last time. We said N equal to 4 super-Yang-Mill theory with [? stage ?] group SU(N) should be the same as the type IIB string in AdS 5 times S5. OK? So, now, other side, now, this is SU(N). And more precisely, this is AdS prime S5 in the Poincare patch. OK? Yes?
AUDIENCE: The N just to recall, is the that N comes from the amount of flux on the S5. Is that correct?
HONG LIU: Yeah, that's right. That's right. So the N here related to the flux on the AdS 5, it's also related, as we will see, related the Newton Constant, et cetera. Yeah, we will see that, yeah. OK?
So now, we can look at this geometric picture. OK? We can look at this geometric picture. Then we observer something interesting. So this SU(N) group, let me say, [INAUDIBLE] R 1,3. OK? On the 3, approximate dimensional-- yeah, let me write it better-- this SU(N) on the space, on the Minkowski space, R 1,3. So now, let's note. Make a simple observation based on the geometry of AdS is that this R 1,3 is actually the boundary of AdS 5. OK? It's essentially the boundary manifold of AdS 5.
And the right hand side, no matter whether you do field theory or string theory, you can always do dimensional reduction on S5. You can always decompose everything on S5 in terms harmonics. So essentially, the right hand side, because S5 is a compact space, the right hand side is a 5 dimensional gravity theory. OK? So we actually now here see a 5 dimensional gravity theory. Now, it's equivalent that your 4 dimensional theory [? lives ?] on its boundary. OK? So this really can be considered [? actually ?] realization of holographic principle.
So actually, there are two ways to think about it. One way is to think from this-- so previously, we motivated. Long time ago, we motivated the duality using two perspec-- [INAUDIBLE]. One is the holographic principle. Some gravity theory should be [INAUDIBLE] to the [? theory ?] living on this boundary. And also, motivated that the Yang-Mills theory in the gauge group SU(N) or U(N), when you do the [? large N ?] expansion, then behaves like a string theory. OK? So this could also be considered as an explicit example of that relation. That this is a Yang-Mill male theory, and then turned out to be [INAUDIBLE] to a string theory. OK?
So now, if you look at this statement, you say, is this a coincidence? Because I don't really see somehow in this picture not so much somehow how why this Yang-Mills theory is actually should be considered as living on the boundary of AdS 5 times S5, because the boundary of AdS 5 times S5, somewhere around here. Yeah, I don't see some Yang-Mills theory living on there, OK? So is this really just some coincidence that just happened to be that?
But actually, there's a very important prediction one can make if you take this point of view. Now, I can erase here. Maybe I will do it here. Yeah, but if you take this point of view, then there's a very important prediction you can make. Then there's a very important prediction you can make, and then check whether that has any chance of working.
So if you believe this picture, then we can have a [? non-trivial ?] prediction, because AdS also have [? a lot ?] of description in terms of the global AdS. Then let's say, what happens if we put the string theory in this global AdS, which certainly, we should be able to do, because these two only differ by some global structure. OK? And this is one part of that. They only differ by some global structure.
So if this is really true, if this is not a coincidence, then we can make a non-trivial prediction is that N equal to 4 is a type IIB string in global AdS 5 times S5. So now, the AdS 5 we take to be the global, to take to be the cylinder. That should be equal to the N equals to 4 super-Yang-Mills theory, which now, should live on the boundary of that cylinder, which is our S3 times R. OK?
So this realization will give you a powerful prediction, which in principle, you can check. OK? And, of course, if this is a coincidence, then there's no reason why this to be true. Because from the brane point of view, we cannot really do the sphere. OK?
AUDIENCE: Why is [INAUDIBLE]? Oh.
HONG LIU: So any questions about this?
AUDIENCE: One question [? on that. ?] so when we derive the right hand picture, we impose that there's some charge on the D-brane. But on the left hand side, it seems that we-- [INAUDIBLE] a the charge [INAUDIBLE]?
HONG LIU: No, the charge is just reflected in open string dynamics.
AUDIENCE: OK. So by your right hand side, we will have the charge.
HONG LIU: No, you essentially have a charge. But in that description, you don't need to introduce a charge. Whatever charge--
AUDIENCE: If we impose charge if the left hand side will never be changed?
HONG LIU: No, no. Just there from the side, you just talk about strings.
AUDIENCE: Uh-huh.
HONG LIU: You don't talk about the whatever charge. The charge is a gravity description.
AUDIENCE: Uh-huh.
HONG LIU: The charge don't even arise in this picture. I just have some strings. I have some open strings. I have some closed strings. They interact with each other.
AUDIENCE: OK. And did this picture go to low energy means it will appear as a charge of the super-Yang-Mills theory.
HONG LIU: No, no, no, no, no. No, no. This goes [? in ?] [? order. ?] You just super-Yang-Mills theory. Yeah. Good? Any other questions? Yes?
AUDIENCE: Is this true?
HONG LIU: Yeah, this is true.
AUDIENCE: OK.
[LAUGHTER]
HONG LIU: And the yeah. So that means this is not a fantasy. Yeah, this tells this is a fantasy. This is actually a powerful realization. This is a powerful realization, yeah. So let me before conclude-- Let me just quickly mention something just in terms of semantics.
So I always say, type IIB string in AdS 5 times S5. So you may ask, does this make sense to say such things? Because if we think this is really as a quantum gravitational theory, so if you have a finite string coupling, then everything fluctuates. And then why we specify a rigid spacetime here? OK? Why do we specify a rigid spacetime here?
So the way you should understand the statement is the following, is that indeed, if you, say, consider the finite G Newton, the finite G string, then the spacetime can fluctuate a lot, will typical be a deviate from AdS 5 times S5. But this AdS 5 times S5 specifies it's asymptotic geometry of AdS. It's asymptotic geometry. It's the geometry of AdS very far away near the boundary.
And essentially, this can be considered as specifying the boundary condition for gravity, the boundary condition for the quantum gravity. So this, essentially, specify the boundary condition. Good, so let's have a couple minutes break. Then we will talk more about the duality.
OK, good. So let's start again.
[SIDE CONVERSATION]
So now, let's move to a new chapter. So now, have we established the duality. So let's try to understand the duality. So let me call it the duality toolbox. And then we can try to use it. So first, let me say some general aspect on the duality. Oh, I erased. Oh, I should keep that figure there.
Anyway, so the first important thing is so-called IR/UV connection. So here, we see the equivalents, say, from the AdS 5 gravity. So we have some AdS 5 gravity to go to N equal to 4 super-Yang-Mills theory in d equal to 4. So from this direction, we may consider as a realization of the holographic principle. OK? We can consider this direction as a holographic principle.
And then you may ask, from the field perspective, if we think from this angle, why somehow, this description of the field theory have one more dimension. So this is a 4 dimensional theory. This is a 5 dimensional theory. OK? What does this actual dimension does? What does this x dimension do, OK? How do we understand from the field theory perspective somehow this increase of one dimension? OK?
So the answer turns out-- the answer is actually very simple. And it's already nice in a way. The answer is already in the way which we take the low energy limit. OK? So remember, when we take the low energy limit-- so let me draw this figure again-- in the gravity side, and this is going to R goes to 0. OK? So by studying the red shift due to the curved spacetime, we show that if you want to go to low energies, then you want to go to smaller and smaller R. OK?
So you want to go to smaller and smaller R if you want to go to [? lower ?] and [? lower ?] emerges. OK? So smaller r, and then give you smaller energy. So we use this argument to decouple this, the other factor. We say, if you want to go to low energy, you take r go to 0 limit. Then there are infinite distance between them.
But the nice thing about infinities is that after you go to infinity, there's still infinite left. OK? So the same argument also applies in AdS, in the AdS part, after you decouple the other stuff. And the AdS part of this argument still applies.
Still, the same thing happens. The same red shift argument applies. So if you want to go to low energies, you want to go to smaller r. OK? And so this immediately tells us that this actual dimension, this r direction, it's precisely the direction, which external to the N equal to 4 super-Yang-Mills theory, transverse to the N equal to 4 super-Yang-Mills theory.
So this [? immediate ?] [? here is ?] this actual dimension, this r, or in this coordinate z-- remember, in this z, z related to that thing just by R squared divided by r, so that r related to that z. So that the r goes to 0 limit, it tells you that that r dimension, r can be considered, this actual dimension can be considered as representing the energy scale of the Yang-Mills theory, OK, or the energy scale of the boundary theory.
So if you want to go to low energy, you go down [? farthest ?] [? route. ?] OK? This is a very, very important point. And this nice into many phenomenon between AdS and the CFT and the disconnection. Very important, and if you have a good understanding, it will give you lots of good intuitions in you understand the relation.
So let me just to elaborate the argument again, now using this metric. OK? OK, so now, we'll go over this red shift argument again, and to use this metric. OK? So again, so the key thing is that the x mu, which you t go to x appear in this metric, is defining the boundary unit. OK? So this defines-- so this defined in the boundary unit. So this is the unit we use in the Yang-Mills theory. OK? So those coordinates are defined in the boundary units.
So now, if we want to talk about energies in some bulk, say, the local proper time, and the length, and the total proper length at some, say, at some z, OK, so at some location z in the bulk is related by the standard relation, which we can read from there. It say d tau should be equal to R, so this local proper time. Local proper time should be equal to R divided by z dt. And the dl, so local length scale, should be a proper length, should be related R z times dx. OK?
OK, just because of this. You can just read it from here. Locally, you can always write this as a Minkowski metric in d plus 1 dimension. And then for the local observer, and this is the local time, local proper time and local proper length. OK?
And then this tells you that you can easily invert this relation. This tells you the energy we observe in the Yang-Mills theory, was just energy we measure in terms of t should be related to the energy, which we measure locally, so all these relation, local energy. And length scale, now d in Yang-Mills theory, is related to the local length scale by this relation. OK? So this is an inverse of that because of the tau and the [? energy. ?] This is just the same as that. OK? Good?
So now, let's consider the same bulk process. OK? So let's consider the same bulk process. Say, for example, this process we are sitting in this classroom, say, supposing we [? leaving ?] AdS. Let's imagine that happens at different values of z, OK say, for the same bulk process, at different z. OK.
Then, of course, the E local, the local energy scale and the local length scale would be the same. OK? So if we consider the different process at different z, the same physical process at different z, in terms of a local observers, it's the same. So E local and the d local does not change. OK?
But if you translate into the Yang-Mills scale, then you find now that the corresponding Yang-Mills energy scale now proportional to 1 over z, OK, because now, we have fixed E local. OK? We have fixed E local. We are changing z. So now, you find at a different location, now corresponding to a different energy scale in the Yang-Mills theory. Say, the d Yang-Mills we will [? portion it ?] to z. OK?
And in particular, for the same process as z equal to 0, if we move this process close to the boundary-- z equal to 0 means close to the boundary-- then the Yang-Mills energy, the corresponding Yang-Mills energy, actually goes to infinity. Under the corresponding Yang-Mills scale, actually goes-- distance scale goes to 0. So that means this is a mapped to some UV process in the Yang-Mills theory. OK?
And but if you take the z goes to infinity, which means you go to interior, far away from the boundary-- so you go to infinity, far away from the boundary, go to interior-- then this E Yang-Mills, then the corresponding Yang-Mills energy scale will go to 0. And the corresponding Yang-Mills length scale will go to infinity. OK?
So this would corresponding to the IR process. So this will be a low-energy process, and [? much ?] distance in the Yang-Mills theory. So there, of course, [? those run ?] into the IR process in the Yang-Mills. OK? And [? this-- ?]
So normally, if you think about the perspective from AdS point of view, when you go to the boundary, means you've got a long distance. So from AdS point of view, this is going to the IR. OK? And then when you go to the infinity, z go to infinity. You are going to the interior in the bulk. So roughly, it's not precisely-- [? if you ?] [? risk, ?] you can think of this actually going to the short distance in the-- not really the short distance, just-- yeah, this name is not very proper, but let's just call it the UV. Just go into the interior of the bulk.
So now, since you see an [? opposite ?] process going on, in the AdS will go to IR. Then from the Yang-Mills theory, corresponding to going to the UV, but in the AdS, you go to interior. And then from the Yang-Mills theory, corresponding to go to the IR low energy. OK? So here, we have something like IR-UV connection. OK?
In particular, I already said in particular once. [LAUGHS] So but I don't have other words. So in particular--
[LAUGHTER]
--if you really consider the typical gravity process, OK, so if you consider the typical gravity process, so not consider string theory, just consider gravity. Typical gravity process, a classical gravity processes, then essentially, the curvature radius defines your scale. OK? So that means that the typical gravity processes, the E local is of order 1 over the curvature scale. And the typical length scale in the bulk, you can also see that it's also the curvature radius.
So in these cases, if you plug this into this relation, then you can see that for the typical bulk process, you can really identify the E Yang-Mills, essentially, just with the radial direction, OK, because this product will be 1. This product will be of order 1. And then you can really just identify the z, the inverse z with the Yang-Mills energy. OK?
So let me just say this in the little bit slide today, in the picture. Maybe I'll do it here, maybe just do it here. So think about this in the picture. So suppose this is a boundary. So this is the picture. This is the picture, which on the back page of the class it said, if you consider some process here, which we draw a cow, which I don't know how to draw a cow here.
[LAUGHTER]
And at some distance, say, here, some value of z here, and similar process as some other value of z, then the corresponding boundary image of them, so this will corresponding to a bigger guy. And this guy will corresponding slightly small guy. OK? Yeah, I hope you understand the picture. Yeah, [? so ?] the further away, the same process in the interior, they corresponding to a process with a larger distance and a lower energy from the field theory perspective. OK?
So now, let me make some remarks. Yeah, I did not show it very well. Is this clear what I mean by this picture? OK, good. Yeah, a more fancy picture is just in the web page of the class.
So now, let me make some remarks. The first remarks is that the AdS, if you, say, calculate the volume of AdS, so calculate the determinant of this guy, square root of the determinant of the metric, and then integrate over the [? O ?] space. Then you find it's divergent. And particularly, it's divergent because of the infinite distance in going to the boundary. OK?
So often, we will, say, put some cut-off, IR cut-off, near the boundary, say, rather than let z go all the way to z equal to 0. We, say, we stop the space at z equal to some [? epsilon. ?] OK? That's the procedure we often do, just as a mathematical convenience.
Because if you go all the way to z equal to 0, then this factor blows up. And then many things become tricky to do. And then we often, in order to get the finite answer, we always put the z-- put the boundary at z equal to some epsilon. And epsilon's some small parameter. OK?
So and for example, this is what you will do in your p set, in one of the p set problem. OK, when we try to-- when you will check this holographic bound. And that's the trick [? you're ?] [? ready ?] to use.
So putting the IR cut-off in AdS, at some z equal to epsilon, then from this IR-UV picture, then translate in the boundary. In the boundary, we introduce a short distance cut-off, or UV cut-off, say, at some short distance scale, say, [? data ?] x say or order epsilon, or energy cut-off, or UV energy cut-off at, say, energy, say, of order 1 over epsilon. OK?
So this just a [? natural-- ?] the reason this goes 1 into a UV cut-off because of this relation, because of this relation. When you cut-off the space at some z, then you can no longer go to infinite energy. You can no longer go to infinite distance scale, OK? And essentially, equivalently providing a short distance cut-off from that relation. OK? Is it clear? And you will use this in your p set. OK?
So the second remark is that here, we are considering, so N equal to 4 super-Yang-Mills theory, as we said, it's a conformal theory. Or it's a scale invariant theory. A feature for scale invariant theory is that there's no scale. There's no scale means you can have arbitrarily low energy excitations. OK?
So for conformal theory, say, in R(1,3), there exists, because of the scale invariants, there exist arbitrarily low energy excitations. OK? So from this IR-UV, then this corresponding to-- then this just map to the z equal to infinity region in the bulk. OK? So this map to the z equal to infinity region in the bulk. So it's a very good thing that this z equal to infinity region. OK? It's a very good thing, this z equal to infinity region, because otherwise, those modes have nothing to map to.
So on the other hand, even the theory have a gap, on the other hand, if, say, if the corresponding bulk spacetime, say, "ends" at the finite proper distance, OK, so important thing is the following. So let's take any point here. Take any point here. So this is some reference scale, say. Choose it as a reference scale in the field theory, because of this [? UV ?] [? R ?] connection.
So now, we want to consider arbitrary low energy scale compared to this scale. Then what you want to do, then you just you go to z equal to infinity. And this, what is actually hidden here when you do this argument, is actually the proper distance going to z equal to infinity is infinite. And because when you do this proper distance, yeah, essentially, there's infinite proper distance [? in ?] go to z equal to infinity. And then you can go to infinite low energy scales. OK?
But now, if you look at the global AdS, then take some interior point. Then actually, you can easily check. When you go to the most interior point, it's just ro equal to 0. OK? And that is finite proper distance away. So in some sense, the spacetime ends at the finite proper distance in the radial direction. OK? In this case, if you apply this, this will tell you that the theory cannot have arbitrary low energy excitations. OK? Is this clear?
Let me just say it again. In this theory, in the Poincare patch, in the bulk, we can go to z equal to infinity. And that's infinite proper distance away. And using this UV IR argument, that translates to infinite low energy process in the field theory.
But now, let's consider what's happening in the global AdS. Just from the geometry, we see something dramatic happens, because now, this is a cylinder. And look at the metric. You take finite distance from some point to go to the most center point, which is rho 0. So you no longer have infinite distance to go to.
So if this argument is consistent, then this must be due to a theory without arbitrary low energy excitation. OK? But this is, indeed, the case, because according to what I erased, just before we break, we say for the gravity theory in the global AdS, that should be due to a Yang-Mills theory, and the boundary over here, which is S times R. For any field theory [? under ?] S-- again, this is a compact manifold-- then there's a mass gap from the vacuum. And so this theory actually have a mass gap. OK?
So that means if the corresponding bulk space that ends this on finite proper distance, the boundary theory must have a mass gap, and vice versa. If the fields theory have a mass gap, which you cannot go to arbitrary low energies, and then the bulk spacetime have to be end somewhere. Otherwise, these statements will not apply. OK?
So consistency check of that statement is that this has to be true. OK? So in the p set, you should think through these for the process over the field theory on the cylinder. OK? OK, I think I will stop here. Do you have any questions regarding this? Yes?
AUDIENCE: I was somewhat under the impression that the global AdS and the Poincare patch were just different coordinates for presentation with the same thing, sort of? So how -- would that be correct?
HONG LIU: Hm?
AUDIENCE: I was under the impression that the global AdS and the Poincare patch are just different coordinate charts on the same sort of structure or [INAUDIBLE].
HONG LIU: Yeah.
AUDIENCE: So why do we have infinite distance in one and finite distance in another? Is that?
HONG LIU: Figured out. Figured it out. And this is geometry. You can just look at them. It depend on how you slice the spacetime. Yeah, it's very easy to see. Just see how you map one point to the other point, et cetera. Yeah.
AUDIENCE: OK.
HONG LIU: But the important thing, the important thing is that the-- yeah, I'll give you a hint. So this z equal to infinity can be considered as a coordinate singularity of that [? thing. ?] so even though here it's completely smooth, but in here is like a coordinate singularity because of this.
AUDIENCE: OK.
HONG LIU: Yeah, yeah, yeah. Yeah, so that's why, actually, to talk about theory on R3 and the theory on S3 is heading [? on 2 ?] because the physics are very different. Because here, yeah, because here, you always have a mass gap. And here, you don't. And so that refract the geometry very different. The way you view the geometry are very different.
AUDIENCE: So the [INAUDIBLE] 5 [INAUDIBLE]?
HONG LIU: Hm?
AUDIENCE: The [INAUDIBLE]?
HONG LIU: Yeah, yeah. [INAUDIBLE]
AUDIENCE: But if you change this [INAUDIBLE], then it's still N equal 4 super-Yang-Mills [INAUDIBLE].
HONG LIU: Yeah, it would be something else.
AUDIENCE: Then yeah.
HONG LIU: Yeah, yeah.
[APPLAUSE]
Free Downloads
Video
- iTunes U (MP4 - 240MB)
- Internet Archive (MP4 - 240MB)
Subtitle
- English - US (SRT)