Flash and JavaScript are required for this feature.
Download the video from iTunes U or the Internet Archive.
Description: In this lecture, Prof. Liu first discusses closed string spectrum obtained from light-cone quantization. The essential point is that among closed string excitation there is a massless spin-2 particle, which can be interpreted as the graviton. He then briefly discusses string interactions which confirms the interpretation of the graviton.
Instructor: Hong Liu
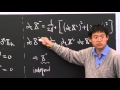
Lecture 12: String Spectrum...
The following content is provided under a Creative Commons license. Your support will help MIT OpenCourseWare continue to offer high quality educational resources for free. To make a donation or to view additional materials from hundreds of MIT courses, visit MIT OpenCourseWare at ocw.mit.edu.
PROFESSOR: Start by reminding you of what we did in last lecture. And, in particular, there was a little bit of confusion. It seems like, maybe some part, I went too fast. So we'll try to-- yeah.
[AUDIO OUT]
So again, we start, say, by choosing the worldsheet metric to be the Minkowski. Then the worldsheet action just reduce to that of a bunch of free scalar fields. Just reduced to a bunch of free scalar fields. And those free scalar field is a bit unusual, because, in particular, there's a 0 component, which we have a long sine kinetic term, OK?
But, of course, this is not our full story. You still have to impose the so-called Virosoro constraint, because [INAUDIBLE], the stress tensor of this series should be 0, which is the consequence of the equation of motion for gamma.
But nevertheless, you can write down the most general solution to this problem, so let me write it here. So the most general of a closed string, or the most general conclusion can be written as x mu plus p mu tau.
So I've now written, what I called previous by Xl and the Xr, I have written them explicitly in terms of the Fourier modes, OK? And I have written them, explicitly, in terms of Fourier modes. And in this form, so you can compare with what we discovered before, previous, here, because v mu, then we discussed last time, that this v mu should be considered as related to the center of mass, momentum, over the whole string. And, for example, for the closed string, that's the relation.
OK, so similarly, for the open string, if you use the Neumann boundary condition, here, use the Neumann boundary condition, then you find, OK, now we'll substitute the explicit expression for the Xl and the Xr. So now, it become 2 alpha prime p mu tau. So, again, this is our previous v mu. So this 2 is related to the open string. Sigma only go from 0 to pi, rather than 2 pi.
And then, you can write the oscillator, this Xl Xr in terms of the explicit Fourier transform. So here, you only have one set of modes. And of course, n sigma just come from you have Xl plus Xr, and Xl, which is equal to Xr. And yeah, when these two become the same, when these two become the same, then these two combine. Because the sigma have opposite sign that combine to cause n sigma. OK? Yeah, it cause a m sigma.
So this is the most general classical solution, and in the light-cone gauge, we say x plus can be setting to 0. And also everything related to alpha plus, and for tilde plus, all the oscillation modes also said to be 0. We raise it to the plus, set it to 0, so there's only [? tau ?] [INAUDIBLE] left in the light-cone gauge.
And Virasoro constraint, they become-- I have tau x minus. So this v plus is the same as that, related to the p plus that way. only single v plus. OK, so you know the equation, so the i should be considered as a sum, OK? A sum of all directions, or transpose directions.
So, from the Virasoro constraint, you can deduce X minus. You can deduce X minus. Yeah, again, let me just write here, our convention's always X mu is equal to X plus, X minus, then Xi. And i goes from 2 to D minus 1, and X plus minus is, say, 1 over square root 2, X0 plus minus X1, OK?
So, from here, you can deduce the X minus. And also, it means that independent variables-- so this, we have determined the X minus up to a constant, because this determines the tau and the sigma derivative up to a constant. So, the independent variables. Xi, and then, also, this p plus, or v plus which appears in the plus, and then the X minus. The 0 modes for the X minus, which is not determined by those things.
And these two, this is just a constant. And, again, these two are constant. These are two-dimensional fields, OK? Any questions so far? Good. So actually, [INAUDIBLE] that the zero mode part of this equation particularly important. Yeah, let me call this equation 1, this equation 2.
So the 0 part of those equations are particularly important. And, for example, say, from equation 1, so the zero modes part, for the first equation-- so let's do it for closed string-- then zero mode part, you just-- alpha prime p minus, OK, you should take the root of alpha tau. So you just get the alpha prime p minus, and the right-hand side.
So, right-hand side, let me also rewrite the v plus in terms of a prime, so this is 2 alpha prime p plus. And then, the zero mode means that you integrate over, you integrate over the string. OK. So this is the first equation. So the zero mode equation become like that.
So, now, let me just make a brief comment, which I, at the beginning, I forgot to mention, last time, which apparently causes some confusion later. It's that, if you look at this expression, this is actually precisely-- so if you look at that two-dimensional field theory, this is actually precisely the Hamiltonian, the classical Hamiltonian for that field theory, OK? For the Xi part.
This is just a free scalar field theory. Yeah, so, in particular-- so let me write that more precise. Let's take out this p plus, and then this become 4 pi alpha prime, and then this just become exactly the Hamiltonian of that theory, because 1 and 2 for the Xi, OK? So H Xi is the Hamiltonian for two-dimensional quantum fields. Quantum field theory of Xi, for the transverse directions, OK?
So you can also write these explicitly in terms of those modes. In terms of those modes, then, for example, you write p minus. Yeah, if you can also write modes, then become p minus. You could, too, 2p plus, pi square, plus 1 over alpha prime.
So if you just substitute those expansion into here, then you can also write it explicitly in terms of modes. OK. And then you can combine-- so this is p minus, then you can multiply this to the other side, combine all the p together. You can write it as M squared, which is defined to be p mu. P mu minus P mu P mu, which is then 2P plus P minus minus Pi squared.
And then, this then become equal to 1 over alpha prime sum m mu equal to 0 alpha minus m i alpha m i plus alpha tilde minus m i alpha tilde m i, OK?
Then, you see that this constraint for p prime, for p minus, now can be written, can be rewritten in terms of the relation of the mass of the whole string in terms of its oscillation modes, OK? In terms of its oscillation modes.
And, similarity, for the open string-- so, this is for the closed string-- for the open, you could act the same thing applies, just you only have, now, one side of modes. So remember, in those expressions, sum over i is always assumed. And whenever I wrote m not equal to 0, it means you always sum for minus infinity plus infinity, and except where m equal to 0, OK?
Yes? Any questions? So these are the consequence of the zero modes for 1. And the consequence for the zero modes for 2, again, you can just integrate over all direction of the string. Then the left-hand side just get 0, because the x minus is a periodic function, so this is a total derivative, and so this is give you exactly 0.
And then, for the constraint, on the right-hand side, on this expression, which can be written explicitly. So you can also, then, plus into the explicit mode, and then they become sum m not equal to 0, alpha minus mi alpha mi equal to [INAUDIBLE].
OK, so this is sometimes called a level matching condition. So this tells you that the oscillation from the left-moving part-- this is for the closed string. For the open string, this equation just does not give you anything. For the closed string, this gives you a non-trivial constraint. Oh, I got a ring.
So, for a closed string, this just tells you that the left-moving part and right-moving part have to be balanced. And this is related to that string. It's periodic. Along the string, it's periodic, so there's no special point on the string. And then, there's no special, and then you can actually not distinguish between the left-moving and the right-moving part, OK?
Good. So this is a the whole classical story. This is, in some sense, the complete classical story, OK? And then, quantum mechanically, we just need to quantize those guys. Quantize those guys. And those are just ordinary quantum mechanic degrees of freedom we don't need to worry about. And this just become a quantization of a free scalar field theory. OK.
STUDENT: [INAUDIBLE] all these modes are massive? Or is there any conditions it can be massless?
PROFESSOR: No, this is a massless field theory. We are quantizing this theory, right? Yeah, so this is massless scalar field theory in two-dimension.
STUDENT: But so that mass is--
PROFESSOR: No, this mass is the mass of the string. No, this is the mass of the center of-- this is the total mass of the string. When I say the massless, this is a massless scalar field theory in the worldsheet. This is a spacetime description. So it's important to separate two things. Things happening on the worldsheet and things happening in spacetime.
So this is the mass of the string viewed as object moving in the spacetime. And when I say quantizing a massless scalar field theory, it's to think of those, the mode. So Xi is saying here, describe the motion of the string. Think of them as a field theory on the worldsheet. And that's a massless field theory.
STUDENT: OK.
PROFESSOR: Right. Yes?
STUDENT: [INAUDIBLE] definition of [INAUDIBLE]?
PROFESSOR: It's the conserve the loss of charge for the string. Cause 1 into the translation. It's to conserve the loss of charge, cause 1 into the translation. Yeah, so that's what we derived last time. So be sure that this v-- but to be original, write v, here-- are related to the center of mass momentum in this particular way. Yes.
STUDENT: Could you use the fact that the right [INAUDIBLE] looks like [INAUDIBLE].
PROFESSOR: Sorry, which one?
STUDENT: The fact that this alpha [INAUDIBLE] P minus is actually the Hamiltonian. Can you use it anyway? Or it's just the--
PROFESSOR: No, this is a remark. And this will make them more lateral. For us, this is not the essential remark, but it will be more lateral, when we work out the zero-point energy. And when we work out zero-point energy, then that's a more lateral thing to consider, because that will actually give you a way of the computing zero-point energy. Yeah. Yes.
STUDENT: So how do you-- [INAUDIBLE]. If you plug in this extension, does it really direct me [INAUDIBLE]?
PROFESSOR: Yeah.
[LAUGHTER]
STUDENT: [INAUDIBLE] n times--
PROFESSOR: Sorry?
STUDENT: Are you supposed to have n time [INAUDIBLE] times the other number of alpha?
PROFESSOR: Sorry, what are you saying?
STUDENT: Here, if you plug in this [INAUDIBLE]. And in the end, you've got m times alpha m
PROFESSOR: Yeah.
STUDENT: But you directly use this equation.
PROFESSOR: Yeah.
STUDENT: And you-- well, [INAUDIBLE]--
PROFESSOR: Oh. This is trivial to see. We can see it immediately here. First, if you take the derivative, we just get Pi, and then you just get that term. And when you take derivative here, then you cancel the m. And then, in order to get the zero modes, then the m and the minus m have to cancel. So the only structure that can happen is this one.
Then, the only thing left remaining is to check the quotient, here, is 1. And that, we have to do the calculation. And the rest, you don't need to do the calculation. Yeah, the only thing to do the calculation is for this one. Yeah.
STUDENT: So just kind of to bore you, so all we're doing is free fields in a two-dimensional worldsheet with the diffeomorphism and variance as a gauge symmetry, and that gauge symmetry gives us some complicated constraints, which we then get rid of.
PROFESSOR: Solve the constraints, yeah.
STUDENT: That's all there is to it.
PROFESSOR: Yeah, that's right. So, as I said before, solving the constraint help you two things. First, you solve this constraint. And second, you get rid of this X plus and X minus. Because those are the dangerous field theory, and now we get rid of. And what's remaining, Xi, are good. Will be, hey, they're good boys. They're good, well-behaved field theories. Any other questions?
STUDENT: Yeah, one question. So isn't knowing how to do, basically, string theory-- well, is it knowing how to deal with the Polyakov action, and not in the light-cone gauge?
PROFESSOR: Oh, sure. But that take much longer time. Even in the light-cone gauge, I'm still trying to telling a long story short.
STUDENT: OK.
And so I'm trying to give you the essence. But make sure-- I'm trying to tell you the essence, but at the same time, I want you to understand. So make sure you ask, whatever, it's not clear.
But, for example, in the next semesters of undergraduate, of string theory for undergraduate, you will reach this point, essentially, after maybe 15 lectures. And we've reached here maybe only two or three lectures. And so, I'm trying to just give you the essence, and to try not to give you too many technical details. Say, cross checks, et cetera. There are many cross checks you can do, et cetera. I'm not going those things. Just give you the essence. Any other questions? Good. OK.
So this is the review of the classical story. So now, let's do the quantum story. Again, this is a quick review of what we did last time. So, quantum mechanically, all those become operators. All those become operators. And the alpha also become operators.
OK, so this commutator, as we said last time, implies that 1 over square root of alpha m i should be considered as standard [INAUDIBLE] operators, for m greater than zero. And the mode will be [INAUDIBLE], should be considered as the creation operators, OK? Creation operators. And then, this is the standard of quantization, or field theory. You reduce to an infinite number of harmonic oscillators.
And in particular, this product just reduced to m times this Ami dagger, Ami just become m Nmi. OK? So Nmi is the oscillator number, occupation number, say, for each harmonic oscillator. Then, the typical state of the system just obtained by acting those things on the vacuum.
For example, for the open string, we only have one set of modes. So then, we would have this form. m1 m1 alpha minus mq, iq, mq on the vacuum. And the vacuum also have a quantum number, p mu, because those p, those p plus and the pi, here, are quantum operators. And so, we take the vacuum to be eigenstate of them. And they're independent of those alphas.
So this vacuum is the only vacuum for the oscillators, but they are still labeled by a spacetime moment, OK? So labeled by the spacetime momentum of the string. So for the open string. And for the closed string, similarly, you just have two sets of modes. Minus i1 n2 alpha minus m2 i2 and 2. And then, also, you have the other. let me call it k1 j1 l1 alpha minus k2 j2 l2, et cetera. In the vacuum. So this is a typical state, for the closed string.
But the allowed state should still satisfy this constraint. OK, should still satisfy this constraint. So this constrain can be written as, reach the following constraint. It said, the oscillator number on the left-hand side, for the left-moving mode, or tilde. And the oscillator number for the right-moving mode, they have to be the same.
So let me rewrite this equation, in terms of oscillator numbers. So this means i sum. So let me, now, write them from m to infinity. So m Nm i. OK, so this just form that equation, OK? Yeah, so this is called the level matching condition.
So, for closed string, you cannot just act arbitrarily alpha and alpha tilde. The number of modes, the total thing you act from the left-hand side, from the alpha and those from the tilde, they have to be balanced by this equation, OK? Which is a consequence that there's no spatial point on the string.
So now, we can also rewrite those equations at the quantum level. OK, so those equations, the quantum level, those equation just tells you that, for those states, the P mu are not arbitrary. P mu are not arbitrary. P mu must satisfy this kind of constraint. P mu must satisfy this kind of constraint.
And that's can, in turn, be integrated as the determine the mass of the string, OK? Determine the mass of the string. So the mass of the string, so then for the open, mass of string can be written 1 over alpha prime sum over i sum over m infinity m Nm i. Then plus some zero-point energy.
So this is just the frequency of each mode. So this is just the frequency of each mode, a frequency of each mode. And then, this is the occupation number. So this is a standard harmonic oscillator result. And then, at the quantum level, of course, there's ordinary issue, because the alpha m and m don't commute. Because [INAUDIBLE] creation, [INAUDIBLE]. So the ordering here matters. So the ordering here matters.
So, in principle, yeah. It matters. Immediately, you can write it. So that will give rise to this zero-point energy, or ordering number. So, similarity, for the closed string, then you just have two sets of modes. Similarly. that's a0.
So a0 can be-- so this is the place this remark is useful, because this just come from-- essentially, this part, just come from, essentially, it's just the Hamiltonian of the Xi. Just the Xi viewed as a field theory on the worldsheet. And the field theory of Xi, essentially, is a bunch of harmonic oscillators. And for each harmonic oscillator, we do know what is the ordinary number. It's just 1/2. Zero-point energy, just 1/2. And then you just add all of them together, OK? Just add all of them together.
So, for example, for the open string, this just become alpha D minus 2. D minus 2 because that D minus 2 derive directions. And then, your sum m equal to infinity 1/2 omega. OK, 1/2 omega, and omega is m.
Then, I told you this beautiful trick last time, that this should be equal to D minus 2 divided by 24 1 over alpha prime, because this guy, the sum over m 1 to infinity m, give you minus 1/12. OK, so this is for the open. So, similarly, for the closed string, you can do the similar thing. Just differ by sum 2, et cetera.
So this give you D minus 2 24 4 divided by alpha prime. Closed. OK? So this vacuum energy-- So this can also be integrated as a vacuum energy on the circle. Vacuum energy of this quantum field theory on the circle.
And, in quantum field theory, this is sometimes called the Casmir energy. And you can check yourself, that those answers agree with the standard expression for the Casmir energy on the circle. Yeah, if you choose here, [INAUDIBLE] property, because we have chosen the sides to be 2 pi.
Good. So this summarized what we did, summarized what we did so far. Any questions on this? Good, no more questions? Everything is crystal clear? I should immediately have a quiz. Yes?
STUDENT: So the 26 dimensions comes from making this 0?
PROFESSOR: No. No, if you put the 26 here, this is not 0.
STUDENT: I'm sorry. I'm thinking 1 over-- I apologize. Not 0. So, you said, the 26 dimensions comes from the fact that, somehow, 26 minus 2 over 24 is 1. It's like it's numerology, but it's like--
PROFESSOR: Yeah. Yeah, that's a very good observation. That's exactly the reason I write in this way. Right. So let me just make a comment. Maybe I make a comment later. Anyway, so from here, from these two expression, from this expression and this expression, you see this picture, which I said at the beginning.
So each of these describe a state of a string. And the state of a string, the state of such a string, they oscillate in this particular way. Say they have those oscillation modes, and then it moves in spacetime, besides your center of mass momentum, besides center of mass momentum.
So such object, you look at it from afar, it's just like a particle, OK? It's just like a particle. So we have established it. So now, let's look at the spectrum. So each state of a string can be considered a map to a spacetime particle. So let's now work out.
And the mass of the particle can be worked out by those formulas. OK, so let's now just work out what are the mightiest particles, because we are interested in the mightiest particles, typically, OK? So now, let's start from the beginning. So now, let's start with open string.
So the lowest mode, of course, is just the vacuum. P mu. OK, there's no oscillators. So, for such a mode, Nm i just equal to 0 for all m and i, OK? So this should be just a spacetime scalar. So this should describe a spacetime scalar, because there's no other quantum number, other than the momentum.
So it should be a spacetime scalar. It should describe a scalar particle. And the mass of the particle, we can just read from here. So the M square equal to minus-- so this is for the open string, so we use this formula. That's the only thing. Because this here is 0. So the only thing come from a 0 term.
So you're just given by D minus 2 divided by 24 1 over alpha prime. So why you need anything in lower case is that this guy is smaller than 0 for D greater than 2. Say, for any spacetime dimension greater than 2, you actually find the mass square, [INAUDIBLE] mass square.
So people actually gave a fancy name for such kind of particle. They call it tachyon. And, in the '60s, actually, people designed an experiment to look for such particles, particles of negative mass, negative mass square. Anyway, we are not going to here.
Let me just say, for the following, in the theory, if you see excitations, if it's a negative mass square, typically, it tells you that the system have instability, that you're not in the lowest energy state. That you are not in a low-energy state.
So what this tells you is that this open string propagate in the flat Minkowski spacetime may not be the lowest configuration of the string, but that's is OK. If you're not in the lowest configuration, it's not a big deal, and it just means you have not found the correct ground state. It does not mean the theory is inconsistent, OK?
And so, even though this is unpleasant, this thing is tolerable, OK? This thing is tolerable. Any problem with this?
STUDENT: [INAUDIBLE]. You already set [INAUDIBLE] m equal to 0, so that is the ground state.
PROFESSOR: No, this is a ground state on the worldsheet. But in the spacetime, this goes one into a particle. This goes one into excitation in the spacetime. And so this goes one into excitation in the spacetime, with an actual mass square.
And, typically, if you have something with a actual mass square-- let me just say one more words, here-- then that means you are sitting on the top of a hill, and that's where you have a actual mass square. And so, it means you are in some kind of unstable state. But, of course, you are allowed to sit on the top of a hill. It's not a big deal.
STUDENT: Why is this unstable, like a [INAUDIBLE].
PROFESSOR: On the top of the hill, is it stable?
STUDENT: No, why [INAUDIBLE] means [? uncomplicated. ?]
PROFESSOR: Oh, if you write a scalar field theory. Yeah, so this goes one into a scalar field in spacetime, now, OK? So now, if I write a scalar field theory in spacetime, say, let me call phi, with a actual mass squared. So that means, the potential for this mass square is like this. Then, that means that the phi wants to increase.
STUDENT: Is that the same thing as example 4, with spontaneous--
PROFESSOR: Yeah, that's right. Similar to 4, [INAUDIBLE]. Except, here, we don't know what is the bottom. We are just sitting on the top. Anyway, so later, we will be able to find the way to get rid of this. So it's OK. So you don't need to worry about this, here.
So the second mode, the second lowest mode, you just add alpha minus 1 on this worldsheet ground state, OK? So now, this thing is interesting. First, this index i, this index i is a spacetime index. It's a spacetime index. And so, this actually means, this transform means this state transform as a vector under So D minus 2 the rotation of the Xi directions.
And, remember, in the light-cone gauge, the [INAUDIBLE] symmetry's broken, and this is the only symmetry which is manifest. And so, that suggests this state should be a spacetime vector, OK? Should be a spacetime vector.
So now, let's work out its mass. So now, m equal to 1. So now, m equal to 1, and so, you just have 1, here. And so, then you just put the 1 here. So this is the alpha prime 1 minus D minus 2 divided by 24. So this is 26 minus D divided by alpha prime. OK, so now you see the 26 divided by 24 alpha prime.
So now, you see this magic number, 26. So now, we emphasized before, in the light-cone gauge, even though only this So D minus 2 is manifest, because you break Lorentz symmetry. The gauge break Lorentz symmetry. But your theory is still, secretly, Lorentz symmetric. It should still be Lorentz [INAUDIBLE], because the string is propagate in the flat Minkowski spacetime.
That means, all your particle spectrum, they must fall into [? representations ?] of the four Lorentz group, OK? They must fall into the [? representations ?] of the four Lorentz group, even though the Lorentz symmetry is not manifest, here. And now, let us recall an important fact.
A Lorentz vector, a vector field. Yeah, just a vector particle. In D Minkowski spacetime, D dimension of Minkowski spacetime, if this particle is massive, then have D minus 1 independent components, so independent modes. And if it's massless, then I have D minus 2 independent modes, OK?
So the situation we have for many of these is the D equal for four, four-dimensional spacetime. So in four-dimensional spacetime, a massless vector is a photon. Photons have two polarizations, have two independent modes. But if you have a massive vector, then you actually have three polarizations, rather than two, OK?
But now, we see a problem. Here, we see a vector, but this factor only have D minus 2 components. Because i-- because these are the only independent modes. Here, we have a vector which has only D minus 2 components. Independent components.
OK, so if you compare with this list-- because there's nothing else. Because these are the only independent modes, here. In the last [INAUDIBLE], there's nothing else. So by compare with our knowledge of the Lorentz symmetry, we conclude the only way this particle can be mathematically consistent, we said, it has to be a massless particle.
So that means M square have to be 0, OK? So M square has to be 0, means that D must be equal to 26. And we actually find massless particles. We actually find the photon. So we actually find the photon in the string excitations. Yeah, one second. Let me just finish this.
For D not equal to 26, Lorentz symmetry is lost. Lorentz symmetry is lost. It's because that means this particle, where M square is not 0, no matter what, these states cannot fall into a [? representation ?] of a Lorentz group. And so, being said, Lorentz symmetry is not maintained, even the Lorentz symmetry is a symmetry of the classical action, but it's not maintained at quantum level.
Somehow, in the quantization procedure, a symmetry which is in your classical theory, it's lost, OK? And this tells you that the quantization is not consistent. It's inconsistent. Because it means, whatever it is, if you have something propagate in Minkowski spacetime, is has two [INAUDIBLE] [? representations ?] of the Lorentz group.
That means that, yeah, this just cannot be the right-- you have to go back, to redo your thing. This is not propagating in the Minkowski spacetime. So, alternatively-- so this is a conclusion that the D must be equal to 26, OK? So you can reach the same conclusion the following way.
So, right now, so the way we did this, we said we fudge this 0. Yeah, we did not fudge it, but we did something to an infinite sum, and find a valid answer. Yeah, we have to do an infinite sum of positive numbers, and then find the active number. And then we find, somehow, there's something. D minus 2 and D minus 2 somehow missing 1. Anyway.
Anyway, but this is actually a deep story. It's not, say, just missing 1, or something like that. So you can reach the same conclusion by doing the following. Say, you put here a 0 as undetermined quotient. And it turns out, the same 0-- yeah, so you can check. So here, it tells you that Lorentz symmetry is lost.
So you can double check this conclusion as follows. So, remember, I said that that classical action is [INAUDIBLE] on the Lorentz symmetry, and then this conserve the loss of charge, because one to the Lorentz transformation. And those charge, they become generators of Lorentz symmetry at the quantum level, just in quantum field theory.
And then, by consistency, those Lorentz-- conserve the Lorentz charges, as a quantum operator, they must satisfy Lorentz algebra, OK? Then, you can check with a general D, and with a general a0. And that Lorentz algebra is only satisfied in the D equal to 26 dimension, and this a0 given by these formulas.
OK, so that will be a rigorous way to derive it. Rigorous way to derive it, but we are not doing it here, because that will take a little bit of time.
STUDENT: Does that also involve the [INAUDIBLE] of the small--
PROFESSOR: Hm?
STUDENT: That [INAUDIBLE] of the [INAUDIBLE], alpha mode?
PROFESSOR: Yeah. Yes. Yeah.
STUDENT: [INAUDIBLE].
PROFESSOR: Sorry?
STUDENT: [INAUDIBLE].
PROFESSOR: No, no, no. You're just assuming some general a0, here. You determine this by requiring that the Lorentz algebra is satisfied.
STUDENT: [INAUDIBLE], in terms of this [INAUDIBLE].
PROFESSOR: Yeah, in terms of alpha. That's right.
STUDENT: Then, [INAUDIBLE].
PROFESSOR: Oh, those commutators are fine. Those commutators are just from standard quantization.
STUDENT: Oh, there's [INAUDIBLE].
PROFESSOR: Sorry, which one over 24? No, forget about 1/24. There's no 1/24. There's no 1/24. You just write a 0, here. It's undetermined constant. And check it by consistency. Determined by consistency.
STUDENT: [INAUDIBLE].
PROFESSOR: Yeah, no. Yes?
STUDENT: [INAUDIBLE] we'll have to work [INAUDIBLE] anyway. So why do we worry so much about [INAUDIBLE]?
PROFESSOR: Sorry?
STUDENT: I mean, we'll have to work D minus 4 dimensions anyway. So then, should we just begin with, like, Minkowski space D is just not correct?
PROFESSOR: Right, yeah. So will find, actually, this conclusion does not depend on the details. It does not depend on details. Yeah, so you can generalize this to more general case. Say, curve spacetime, et cetera. And the [INAUDIBLE] spacetime [INAUDIBLE]-- then, you'll find the same conclusion will happen. The same conclusion will happen.
And then, you reduce to four dimensions, then you will find the fourth dimension a massive vector which only have two polarizations. Yes?
STUDENT: Maybe related to this question. We have no evidence that Lorentz symmetry holds in the compactified dimensions. So why do we want to keep it there?
PROFESSOR: We just say, doesn't matter. It's only a question as far as you have some uncompact directions. As far as you have some Lorentz directions, then this will apply. Yes.
STUDENT: Kind of going back a little bit to the [INAUDIBLE] thing. How do we know for a fact that the string tension causes-- because, for example, I think, in QCD strings, isn't string tension negative?
PROFESSOR: Not really. How do you define a negative string tension?
STUDENT: A negative tension? I don't know. I just read that, the QCD string, they have negative string tension.
PROFESSOR: No, I think, here-- so alpha prime is a scale. It's a physical scale. Tension is-- it's defined to be positive. Just by definition, it's positive. Yeah. Other questions? OK, so let me just say a little bit more regarding-- so now we have found a tachyon and a massless vector, and, also, we have fixed the spacetime dimension to be 26. We have fixed spacetime dimension to 26.
So now, if you now fix 0 equal to 26, then the higher excitations are all massive. OK, so for the photon, essentially, it's this guy. So this guy's inactive. This guy cancel this guy. So, when you go to higher excitation, then this guy will dominate, and the m square will be all positive. And the scale is controlled by this 1 over alpha prime, OK?
So will be all massive with spacing, given by, say, 1 over alpha prime. So, we said, spacing m squared given by 1 over alpha prime. For example, the next level would be-- so alpha minus 1 alpha minus 1 some i some j, or alpha minus 2 i acting on 0, P, OK? So those things acting on-- to avoid confusion, let me write it more clearly.
So this acting on 0, P, and alpha minus 2 [INAUDIBLE] on 0, P. OK? So this would be like a tensor, because these have two index. And this, again, like a vector. Again, like a vector. So those would be, obviously, the mass square of 1 over alpha prime. And you can check that, actually, they actually fall into the four [INAUDIBLE] [? representations ?] of the Lorentz group, OK? For [INAUDIBLE] [? representation ?] of the Lorentz group. Yes?
STUDENT: Where is the one with only one index [INAUDIBLE]?
PROFESSOR: Sorry. say it again?
STUDENT: So the one with one index, since it's massive, it's supposed to have P minus 1 degrees of freedom, right?
PROFESSOR: They have-- oh. What's happening-- that's a very good question-- so, what's happening is that this should give a tensor [? representation, ?] but this not enough. And this acts together to form a tensor [? representation. ?] Yeah, because i only going from to 2 to D minus 1, so you need to add them together. Good.
So just to summarize story for the open string, we find the tachyon. We find the massless vector, which can be integrated, maybe, as a photon. And then, you find lots of massive particles, infinite number of massive particles, OK? So any other questions, or do you want to have a break? We are a little bit out of time. Yeah, maybe let me give you three minutes break. Yeah, let's have a break, now.
So, again, the lowest state is just the 0, P. And then, again, all the N is 0. All the N are 0. So we just read the answer from here. Then M square, for the closed string, you just equal to a0 for the closed string. So, here, is now m squared minus 4 divided by alpha prime. D minus 2 divide 24.
So, again, this is tachyonic for D greater than 2, OK? Tachyonic for D greater than 2, and this is a scalar. And this is a scalar, because there's no other quantum number. Yeah, so now we are familiar with these tachyons, so we don't need to worry about it. So let's look at the next.
So, next, naively, you may say, let's do this one as open string case, but this is not allowed. This is not allowed. Why?
STUDENT: [INAUDIBLE].
PROFESSOR: That's right. It does not satisfy this condition. Because, this one, you only have the left-moving excitations, but does not have the right-moving excitations. You are not balanced. So you also need to add-- so the next one will be this guy, OK?
So, now-- oh, here, have a j. Now have a j. So this, we'll have m squared 26 minus D divided by 20 alpha 4 alpha prime. 6 alpha prime.
Again, now, look for what representations of the Lorentz group will give you this. OK, you'll find none, unless you're in the D equal to 26, OK? The same story happens, again, only for D equal to 26, fall into the representations of Lorentz group. And reach the m squared, again, it's massless. m square is massless.
So, it turns out, actually, this does not transform under any reducible [? representation ?] of a Lorentz group. It's actually a reducible, so it can be separated into several subsets. So this can be further decomposed to--
So you can take all the i and j together, take the same i, some i. So take this guy, take these two index to be the same, and the sum of all the directions. So this does not transform under the rotation of i's, so this is a scalar. But it's a massless scalar.
And you can also have the situation-- so all the state are in this [? representation ?] of both states, so they are D minus 2. So there are D minus 2 times D minus 2 of them. D minus 2 times D minus 2 of them. So this, D minus 2, say, one of them can be decomposing to a scalar. And I can also take the linear slope [INAUDIBLE] of them with a symmetric traceless.
So the trace part is, essentially, this scalar. And I can also take a traceless part. Traceless e i j, OK, because the trace part is already covered by this one. I don't want to repeat. And this is precisely the generalization, what we normally call the spin-2 representation, to general dimension. OK, so we have found a massless spin-2 particle. So this is a massless spin-2 particle.
And then, you can also, of course, take it to be antisymmetric. So that's the only possibility, now. bij, antisymmetric bij. So these are called antisymmetric. So these will give rise to an antisymmetric tensor in spacetime. So this is an object with a 2 index, and the 2 index are antisymmetric, OK?
So, similarly, the higher modes are all massive. For example, at the next level, next mass level, m square is equal to 4 divided by alpha prime. Any questions on this?
STUDENT: This antisymmetric tensor, what is that?
STUDENT: It's like a [INAUDIBLE].
PROFESSOR: It's a antisymmetric tensor.
STUDENT: But what's the spin?
PROFESSOR: Yeah, normally, in the fourth dimension will be something what we normally call 1 comma 1, a representation of the Lorentz group. Yeah, it's not 0, 2. It's what we normally call 1 comma 1, yeah. Yes.
STUDENT: It's a form of bij [INAUDIBLE]?
PROFESSOR: No, this is just arbitrary. Yeah, so this is your state space, at this level, right? And so, the general state would be [INAUDIBLE] of them. And those states, they transform separately, on the Lorentz symmetry. So we separate them. And so, for example, symmetric strings, they transform separately on the Lorentz transformation under these guys.
So this should cause 1 into different spacetime fields. So each of those things should correspond to a spacetime field, OK? So, now, let me just summarize what we have found.
So we have found-- so let's collect the massless excitations we've found. Because, as we will see, the massless excitations are the most important one. Let me also mention, let me just emphasize. In physics, it's always massless particle give you something interesting, OK?
For example, here, even for D not equal to 26, those massive particles-- as I said, maybe I did not emphasize-- even for D not equal to 26, these massive particles that do form into representations or Lorentz symmetry, for any dimension, only for those massless particles, OK? It almost seems funny.
And so, of course, we also know the massless [INAUDIBLE] that give rise to long-range [INAUDIBLE], et cetera. So now, let's say, let's collect the massless particles. Massless excitations. So we will write them in terms of the spacetime fields. So, for the open string, essentially, we find the massless vector field.
So this is our photon. So, at the moment, I put it as a, quote, photon, because we only find the massless particle. We don't know whether this is our own beloved photon, yet. And, for the closed string, then we find a symmetric tensor. So this is what we normally call the graviton. So again, quoted.
We find the massless spin-2 particle, which, if you write in terms of field, would be like a symmetric tensor, Or B mu mu, which is antisymmetric tensor. And now, this mu mu, this all wrong in all spacetime dimensions, OK? And then we have a phi. Then you have a scalar field.
So this is just called antisymmetric tensor, and this phi is called a [INAUDIBLE]. Phi, which is that scalar field, is often called a [INAUDIBLE].
So, so far, even we call them photon, call this one photon, and the h mu, they are not-- to call them photon and the graviton is, actually, a little bit cheating, because we don't know whether they really behave like a photon or like a graviton, OK?
We just find a massless spin-1 particle and a massless spin-2 particle. But, actually, there is something very general one can say, just from general principle. Just from general principle, one can show, based on Lorentz covariance, and, say, an absence of [INAUDIBLE] physical states, et cetera, say [INAUDIBLE], et cetera, just based on those general principle, one can argue that, at the low energies, that the dynamics of any massless vector field should be Maxwell. And, for the massless spin-2 particle, must be Einstein gravity, OK?
So that's why, say, tomorrow, supposing if you mend this theory yourself, and suppose that's a quantum theory, and that theory just happens to have a massive spin-2 particle, then you don't have to do calculations. Then you say, if my theory is consistent, this spin-2 particle must behave like a graviton, OK?
STUDENT: Are you saying that there's no other Lorentz invariant [INAUDIBLE]?
PROFESSOR: Yeah, essentially, you can show, the low energies, it's always just based on gauge symmetry, et cetera. The only thing you can have is [INAUDIBLE], yeah, is Einstein gravity. Good. And this can be, actually, checked explicitly. So now, let me erase those things, now. Don't need them.
So this can actually be checked explicitly. So, in string theory, not only can you find the spectrum, you can also compute the scattering amplitude among those particles. OK, so I said [INAUDIBLE] before, essentially, perform path integrals with some initial string states going to some final string states, et cetera, OK?
So, of course, this will be too far for us, so we will not go into that. Let me just tell you the answer. So you can confirm this. So this expectation, this confirmed. This confirmed by explicit string theory calculation of scattering of these particles, OK?
For example, let's consider-- so we have this massless spin-2 particle, which I called h. So let's consider, you start with initial state with two h, then scatter to get its own two final stage, which, again, h. So this is, say, graviton graviton scattering. Start with two graviton, scatter them.
So, as in string theory, we'll be, say, at the lowest order, we will have a diagram like this. So a nice [INAUDIBLE] order. I'm not drawing very well. Anyway, I hope this is clear.
So you start with two initial string states. You scatter into some final states, OK? So this is an obvious string theory scattering diagram. You can, in principle, compute this using path integral, which I outlined earlier. Of course, we will not compute this path integral.
And so, you see, there are two vertex here. One is proportionate to string. You have two string merging to one string, and then you have a string that's split into two. So they're two. Remember, each of [INAUDIBLE] equals 1 and 2 [INAUDIBLE] string, OK? So this will be the process, in string theory. So this will be the process in string theory.
So now, If you go to low energies, low energies means that, if you can see that the energy of the initial and the final particles to be much, much smaller than 1 over alpha prime. So, remember, 1 over alpha prime, it's the scale which go from massless to massive particles.
So, if you can see the very low-energy process, which E is much, much smaller than 1 over alpha prime, then the contribution of the mass-- so, in some sense, in the string, in this intermediate channel, when you go from 2 initial state to 2 final state, this intermediate channel, the infinite number of string states can participate in this intermediate process.
But, in the process, if your energy's sufficiently low, then, from your common sense, we can do a calculation. And then, the contribution of the massive state becomes, actually, not important. So, essentially, what is important is those massless particles propagating between them.
And then, you can show that it's actually just precisely reduced to the Einstein gravity. Precisely reduced to Einstein gravity. So more explicitly-- so yeah, so when you go to low energies, then only massless modes exchange, dominate. Terminates. And then, you find that the answer is precisely agreed in the [INAUDIBLE] limit. Agree with that from Einstein gravity.
Because you not only have graviton, you also have this B and phi, they are also massless modes. So this is a slight generalization of Einstein gravity. It's Einstein gravity coupled to such B and the phi, OK? So, in fact, you can write down the so-called low-energy effective action.
So-called low-energy effective action, and we call LEE, here. let me see, like this. So phi is this phi, here, and R is the standard reach scalar for the Einstein gravity. H is this reindexed tensor formed out of B.
So H square just a kinetic term for B. So, more precisely, you can show that the scattering amplitude you obtained from string theory, then you take a low-energy limit, that answer precisely the same as the scattering amplitude you calculated from this theory, say, expanded around Minkowski spacetime, OK? So this is Einstein gravity coupled to [? home ?] scalar field, OK? Yes?
STUDENT: So what about higher loops or higher energies [INAUDIBLE]?
PROFESSOR: Of course, then, it will not be the same. This is low-energies, OK? So let me make one more remark. Make one more remark. In Einstein gravity, say, like this. So Einstein gravity coupled to the matter field. So, when I say Einstein gravity, I always imply Einstein gravity plus some other matter field which you can add.
So, in Einstein gravity, start your scattering process. It's that lowest order, we all know, is proportional to G Newton . OK, so this is the same G Newton, the G newton observed. Yeah, let me call this, say, scattering for this is A4. And then, the scattering for the Einstein gravity is proportionate to G Newton.
So one graviton is changed, and it's proportionate to Newton constant. This is an attractive force between two objects. And, if you look at this string diagram, then this string diagram is proportional to gs square. So we conclude that the relation between the Newton constant and the string coupling must be G Newton proportional to gs square, up to some, say, dimensional numbers or some numerical factors.
So this is very important relation you should always keep in mind. So now, here's the important point. [INAUDIBLE] just asked, what happens at the loop levels? So you can compare the three-level processes, because, actually, find they agree very well. We say, what happens at loop levels?
So now, let me call this equation 1. So, at loop level, this 1 is notoriously divergent. So if you can calculate some scattering amplitude to the loop level, then find the results are divergent. In particular, more and more divergent when you go to more and more higher loops, OK? More divergent at higher orders in [INAUDIBLE] series, say, at the higher loops.
So that's what we normally mean, say a theory is non-realizable. So this tells you-- so, if you take this gravity theory-- so this is just, essentially, our Einstein gravity coupled to some fields-- if you take the Einstein gravity, expand the long flat space, quantize that spin-2 excitations, then that will fail. Because, at certain point, you don't know what you are doing, because you get all divergences, which you cannot renormalize. OK, you can normalize.
So, of course, what this tells you is that this equation itself likely does not describe the right UV physics. So that's why you see all these divergences, because you maybe lost some more important physics, which you cannot renormalize. But now, so this is supposed to only agree with the string theory at low energies, which the maximum modes are not important.
But, in string theory, there are this infinite number if massive modes, et cetera. So you find, in string theory, if you do similar loop calculating string theory, the string loop diagrams, magically, are all UV finite. Or UV finite, so there's no such divergences. There's no such divergences.
So this is the first hint. So this was the first hint of string theory as a consistent theory of gravity. And because, at least, at the pertubative level, you can really quantize massive spin-2 particles, and to calculate their physics in the self-consistant way, OK? Any questions on this? Yes.
STUDENT: In that pertubation, you'd have to use all those upper--
PROFESSOR: Yeah, that's crucial. So that's why this kind of thing is not good enough, because that does not have enough degrees freedom. So, in string theory, you have all these additional degrees of freedom that make your UV structure completely difference. Yes.
STUDENT: Then if you take that [INAUDIBLE] and you just [INAUDIBLE] from above on [INAUDIBLE], will it make [INAUDIBLE]?
PROFESSOR: Yeah, so this will work, as what normally works as a low-energy effective theory. A low-energy [INAUDIBLE]. When you consistently integrate out the massive modes. And yeah, so, even at loop level, this can capture, indeed, at low energies, in my loop level, this can capture some of the string theory with that. But you have to normalize properly, et cetera, yeah. Yes.
STUDENT: Does this imply that, for large objects, not things on these very small scales, that we should reproduce the Einstein field questions?
PROFESSOR: Yeah.
STUDENT: This is sufficient to show that?
PROFESSOR: Yeah, that's what it tells you. Yeah, for example, if you measure the gravity between you and me, you won't see the difference. Yeah, actually, you will see a difference. So this theory is a little bit different, because of this massive scalar field.
So, in ordinary gravity, the attractive force between you and me just come from graviton. But in this theory, because this scalar is massless and, actually, have additional attractive force. And so, this theory is, actually, not the same. So this theory, even though it's very similar generalization of Einstein gravity, but actually give you a different gravity force.
So that's why, with the string theory, each going to describe the real life, somehow the scalar field has to become massive. Some other mechanism has to make the scalar field massive.
STUDENT: I see.
PROFESSOR: Yeah, of course, we also don't observe B mu m and this also, obviously, become massive.
STUDENT: And will we find out that's the mechanism to make [INAUDIBLE]?
PROFESSOR: Yeah. So this is one of the very important questions, since early days of string theory. People have been trying to look for all kinds of mechanisms to do it, et cetera, yeah.
STUDENT: So there is an agreed-upon way of doing this? Or is it still sort of [INAUDIBLE]?
PROFESSOR: It depend, yeah. This goes to a Lex point. Yeah, wait for my Lex point. Yes.
STUDENT: Can I ask, how do we know that this effective theory couples all the fields as Einstein's theory does, though quandrant derivative?
PROFESSOR: So what do you mean? You can add-- the coupling between them, this and gravity is through the-- Yeah, here, of course, you should use quadrant derivative. Yeah. Yeah, here, I did not-- I'm not very careful in defining this, but here, you use quadrant derivative, et cetera.
STUDENT: What about coupling to the open strings, or to photons, or to matter?
PROFESSOR: Would be the same thing.
STUDENT: As?
PROFESSOR: As what you would expect, that [INAUDIBLE]. Just saying, it just governed by general covariance. General covariance have to arise at low energies.
STUDENT: So that general principle is effective including matter, as well?
PROFESSOR: Yeah, then you can check. They can check. It it's a string theory, anything you can check is consistent with that principle. Good. So now, it's another point. So let me just make a side remark on the physical consequence of this scales field. So we see that this scalar field is important, because it, actually, can mediate, say, attractive force.
But, actually, there's another very important role of this scalar field task. It's that, if you look at this low-energy effective action-- so let me now write this G Newton as 1 over g string square. Then this have the structure proportional to 1 over g string square times exponential minus 2 phi, OK.
So now, there's a very important thing. So now you see, if phi behave non-trivially-- this is, actually, modify this guy-- it's actually factively-- yeah, because this is multiplied by the Einstein scalar-- so this, effectively, modifies your Newton constant, OK? In fact, they modify the Newton constant.
In fact, you can actually integrate this gs as the expectation value of these phi, OK? That's the expectation value of this phi. Yeah, because if you can change your phi, and then change effective gs, then the gs can reintegrate as expectation value of phi.
So this is something very important and very deep, because, remember, gs is, essentially, The only parameter in string theory. The only dimension is parameter in string theory, which characterize the strings of the string. And now, we see this constant is not arbitrary. It's actually determined by some dynamic field, OK?
So that actually means that, string theory, there's no free parameter. There's no free dimensionless parameter. Everything, in some sense, determined by dynamics. So this is a very remarkable feature.
So this makes people think, in the early days of string theory, that you actually may be able to derive the mass of the electron. Because there's no free parameter in string theory, so you should be able to derive everything from first principle. Anyway, but this also create a problem for the issue I just mentioned. Again, this is a side remark, but it's a fun remark.
But string theory, we mentioned before, is a summation of a topology. And the topology's weighted by g string. So, if g string is small, they you only need to look at the lowest topology, because the higher topology are suppressed by higher power of g string, OK?
And, particularly, if g string become [INAUDIBLE] 1, then, to calculate such a scattering, then you need to sum if all plausible topologies, and then that would be unmanageable problem, which we don't know how to do. And so, want g string to be very small, so that, actually, we can actually control this theory, OK?
But now, we have a difficulty. But we also said we want the scalar field to to develop a mass. We want this scalar field to develop a mass. And, turns out, this is actually not easy, to arrange this scalar field to develop a mass, and, at the same time, to make this expectation to be very small. K. And, actually, that turns out to be a non-trivial problem. Yeah, so it's actually not that easy.
OK, so my last comment. So, earlier, we said, the tachyon. So what we described so far, these are called the bosonic string, because we only have bosons. We only have bosons. It's called the bosonic string.
So this bosonic string can be generalized to what's called a superstring theory. Of course, superstring. So what superstring does is the following. After you fix this gauge, again, the superstring can be written as some covariant worldsheet theory with some intrinsic metric.
And, in a superstring, after you fix this gauge, the worldsheet metric to be Minkowski, then the superstring action can be written as the following. You just, essentially, have the previously free scalar action. But then, you add some fermions. You add some fermions.
So these are just some two-dimensional fermions living on the worldsheet. Yeah, so these are two-dimensional spinner fields living on the worldsheet. So the reason you can add such a thing, because those things don't have obvious geometric interpretation. So you can consider them as describe some internal degrees of freedom of the string.
And so, these guys provide the spacetime in the interpretation of moving the spacetime. And those are just some additional internal degrees of freedom on the worldsheet. It turns out, by adding these fermions, actually, things change a lot. Actually, they do not change very much.
It turns out, things does not change very much, because this is a free fermion series, also very easy to quantize. And everything we did before just carry over, except you need to add those fermions You need to also quantize those free fermions. Turns
Out, when you do that, there are actually two different quantization scheme, quantization procedure, quantization process. Two different quantizations exist. So, when you add these fermions with no tachyon. So, you actually can get rid of tachyon by including these fermions, OK?
So then, the lowest mode is just your massless mode, OK? And the reason that you can have more than one quantization is that, when you have fermions-- and this is fermion defined on the circle. So fermion on the circle, you can define to be periodic or antiperiodic.
So now, you have some choices. And, depending on whether you chose fermion to be periodic, antiperiodic, et cetera, and then the story become different, OK? And, sorry, we're not going into there. But, in principle, just waste enough time, in principle, now, you can do it yourself. Because, just quantize free field theory.
Actually, you still cannot do it yourself. Yeah, this there's still a little bit more subtlety than that, but the principle is very similar. So, in this case, you get rid of tachyon. So these two procedures cause type IIA and type IIB string. So they give you two type of string theory. One is called type IIA, and one is called type IIB.
So, also, a lot of the important difference, instead of D equal to 26, now only requires D equal to 10, OK? So now, let me just write down the massive spectrum. So now, because you have fermions, now, actually, this spacetime particle can also have fermions.
Previously, it's all bosonic particles. Now, by adding these worldsheet fermions, it turns out that these can also generate the spacetime fermions. It can generate spacetime fermions. So now, the massive spectrum. So now, these are the lowest particles. There's no tachyon.
So these, now, become all 10 dimensional fields. So for type IIA, again, you have this graviton, this B mu mu, then you have this theta [INAUDIBLE]. And then you have a lot of active fields come from the closed string. And so, this is for the closed string. Now, you actually have a gauge field. Also, in the closed string, you want gauge field and the three form tensor fields. So you have three indexes, 40 antisymmetric.
STUDENT: Is the gauge field just coming from the fermion bilinear?
PROFESSOR: Yeah, yeah, yeah. Right. That's right. They are related, actually, to fermion bilinears, yeah. So those things are exactly the same as before, and then you get some additional fields. So these are normally called the Ramond-Ramond fields. And then, plus fermions. So let me write down the fermions.
It turns out that, these theories, actually was magic. So, each of those fields, they have some super fermionic part So it's actually a supersymmetric theory. So then, you also have type IIB. And, again, the bosonic string, you have H mu mu, B mu mu, phi. And then, you have the larger scalar field, chi, then the larger antisymmetric tensor C mu mu 2, and it's in the four form field.
OK, so this, again, is so-called the Ramond-Ramond. Then plus fermions. And then, the [INAUDIBLE] theory of them becomes, so-called, type IIA and type IIB supergravity. So these are supersymmetric theories, and then the corresponding generation of gravity for the supergravity. So we do not need to go in to there. Yes.
STUDENT: So, if you were going to do string theory, for example, for a the generalization on membranes, as you had mentioned before, if you were doing this on two manifolds, would you also have to include anion contributions?
PROFESSOR: You may. I don't know. Nobody have succeeded in doing this. Yeah, you may. OK, actually, today I have to be particularly slow, because I have a much more grand plan for today.
That also means that there's definitely-- you can only do the first two problems in your pset. There's only four problems in your pset, but you can only do the first two. So you want to start, either, the first two, or you want to wait until next week to do it all together.
STUDENT: If we do it all together next week, so how about the next homework? [INAUDIBLE] one week later?
PROFESSOR: Yeah. OK, yeah, then let's just defer to one week. Yeah, next Friday. Yeah, then the next time-- so these, in sum, conclude our basic discussion of the string theory. You have seen most of the magics of string theory, even though it's very fast. So, next time, we will talk about D-branes, which is another piece of magic from string theory. Yeah.
Free Downloads
Video
- iTunes U (MP4 - 233MB)
- Internet Archive (MP4 - 233MB)
Subtitle
- English - US (SRT)