Flash and JavaScript are required for this feature.
Download the video from iTunes U or the Internet Archive.
Description: This session covers bone and trabecular bone, and begins discussing osteoporosis.
Instructor: Lorna Gibson
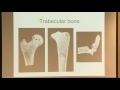
Lecture 11: Trabecular Bone...
The following content is provided under a Creative Commons license. Your support will help MIT OpenCourseWare continue to offer high quality educational resources for free. To make a donation or view additional materials from hundreds of MIT courses, visit MIT OpenCourseWare at ocw.mit.edu.
LORNA GIBSON: So we're going to start talking about trabecular bone, and we're going to do a bone today and on Wednesday. And I'm hoping we can more or less finish it on Wednesday. So hello. Hold on a sec.
So these are some images of trabecular bone, and you can see that it has a foam-like structure. And trabecular bone exists in certain places in the body. There's three main places that it exists. So it exists at the end of the long bones.
And over here this is a femur. This is the very top of the femur, and you can see this is all trabecular bone in here. This is a tibia, and this is the top of your knee there. And you can see how the bone get more bulbous at the ends, and it's filled with a trabecular bone.
This is a vertebrae. So vertebrae are actually mostly trabecular bone, and they have a really thin shell of what's called cortical bone, the dense bone, on top of it. So trabecular bone exists at the ends of the long bones, it exists in the core of the vertebrae, and it also exists in sort of shell or plate-like bones. So in your skull, for example, there's a layer of trabecular bone in between two layers of the compact dense bone. And in your pelvis, it's the same thing.
So those of you who took 3032, you remember when I passed around the bird skulls, there was that very porous kind of trabecular bone. So trabecular bone is of interest medically in three main kind of medical situations. So the first one is osteoporosis. So I want to talk a little bit about osteoporosis now, and then we'll talk about it in more detail later on. I guess, we'll probably start today.
Another medical issue is osteoarthritis, and the properties of the trabecular bone are important in arthritis, and the third issue is in joint replacements. And so we're going to talk a little bit about osteoporosis, osteoarthritis, and then joint replacements. And then I'll talk a little bit more about modeling bone like a foam and how it deforms and how it fails. And then we'll talk a little bit how we can model osteoporosis.
So let me write down some of these things. I guess we'll start here. So trabecular bone has a foam-like structure, and what we're going to see is that we can use the models for foams to describe the mechanical behavior of the bone. It exists at the ends of the long bones. And at the ends of the long bones, the bones become more bulbous.
And really what that's for is to increase the surface area so that there's cartilage between the ends of the two bones. So there would be a bone here and a bone there, and there's cartilage in between them that sort of lubricates that joint and makes low friction at the joint. And the bone gets larger to decrease the stresses on the cartilage. So if you have the same force and you have a larger area, you're going to have a smaller stress. So that's why the bone gets bulbous like that. And then by having the trabecular bone, because it's so porous and lightweight, you're not having a big, dense hunk of bone at the end of the long bones.
So it exists at the ends of the long bones. And I'll just say the ends have a larger area than the shafts. And that's to distribute the loads on the cartilage or to reduce the stress on the cartilage. And then the trabecular bone reduces the weight.
So it also exists in the core of the vertebrae, and in fact, it makes up most of the vertebrae and then in things like the skull and the pelvic bones in shell and plate-like bones. And so it's the core of a sandwich structure there. So it's of interest in osteoporosis, in osteoarthritis, and in joint replacements.
So if we start by thinking about osteoporosis, you probably know that osteoporosis is a disease where the bone mass becomes reduced and there's a greater risk of fracture, so there's especially a greater risk of hip fractures and vertebral fractures. And it turns out in both of those sites, if you just look at these bones here, typically if you have a hip fracture, what happens is the neck of the femur breaks. So this is called the neck here. This is called the head, this spherical bit there.
So the neck has a fracture, and you can see most of the bone there is trabecular bone, so it's really carrying most of the load and the same with the vertebrae. This sort of cylindrical part of the vertebrae here carries most of the load. It has a shell of really thin cortical bone, but it's mostly trabecular bone. And when the loads are vertical like this, that's really the trabecular bone that's carrying most of the load.
And people get sometimes what are called wedge fractures where instead of having a sort of a cylinder with parallel faces like this, the trabecular bone fails, and the bone ends up like that so that there's-- yeah, I know. You make that wincing expression. It's like ouchy. And in fact, it's very ouchy for people who get that.
And when you see little old ladies who are all hunched over, that's why. The bone has actually failed. It's actually been crushed into these wedge fractures, and there's no way they can straighten it out, and it's quite painful.
So people who look at osteoporosis are quite interested in the mechanical properties of trabecular bone for this sort of reason. The hip fractures are particularly serious because people become immobilized and then sometimes because they're immobilized, they get pneumonia, and in elderly people they sometimes die. So something like 40% of elderly patients who are over 65 die within a year of having hip fracture.
So it's not that the hip fracture kills them, it's that they become so immobile, and they can't move, and they can't walk around, and they end up getting pneumonia. So it's quite a serious thing. And there's something like 300,000 hip fractures a year in the US, and the cost of treating these hip fractures is something like $19 billion. So yeah, it's a huge problem. And as the population is aging, as baby boomers like me get older and older, there's going more people having hip fractures. So it's a huge deal, osteoporosis.
So we'll say bone mass decreases with age, and osteoporosis is extreme bone loss. And a little later today I'll show you some pictures of what it looks like when people have osteoporosis. So the most common fractures are of the hip and the vertebrae, and at both sites, most of the load is carried by the trabecular bone.
And the hip fractures you are the most serious. 40% of elderly patients pass away within a year. So that's sort of a little introduction to osteoporosis.
The next issue that people are interested in is osteoarthritis. And in osteoarthritis, there's a degradation of cartilage at the joints, and the stress on the cartilage is affected by the modulus of the bone that presses against the cartilage. You can kind of magic if you have a fiber compass, for instance, most of the stresses, if you're loading it along the fibers, is carried by the fibers because they're stiffer.
So if you have like say trabecular bone that has varying density, the denser bits are going to have higher moduli, and it's there's going more stress associated with that. And so the modulus of the trabecular bone can affect how the loads are distributed in the cartilage, and that can affect the damage in the cartilage.
And the shell, as I mentioned before, this sort of shell of cortical bone or the dense bone at the joints can be quite thin. It can be less than a millimeter. So I brought my little bones along with me again. So this is the head of a femur here, and this is a piece of a knee joint here from a tibia. And you can see just looking at these how thin the cortical shell is. So you can get an idea of how thin that is.
So osteoarthritis involves a degradation of the cartilage at the joints. And the stress on the cartilage is affected by the moduli of the underlying bone, and the cortical shell, the totally dense bone, can be quite thin. So the mechanical properties of the trabecular bone can affect the stress distribution on the cartilage.
And if osteoarthritis gets particularly bad, then sometimes people have joint replacements. So when it gets really bad, the cartilage is degraded completely, and the bone is rubbing on bone, and that's quite painful. And when it gets to that point, people generally have a joint replacement.
And so the way the joint replacements are done is say somebody who is going to have a hip replacement, what they do is they chop off the top of the femur. So they would chop the femur off somewhere around here, and then they have a metal implant that has a spherical ball. That's like the head of the femur. And then it has a sort of stem and a shaft here that goes into the hollow part of the long part of the shaft of the femur.
And so they use a number of different metals for this, titanium and stainless steel, and there's a cobalt-chromium alloy are also used. So you need metals that are biocompatible, aren't going to corrode, aren't going to have degradation products. And then the bone grows around that implant, and the bone grows in response to mechanical loads. So the density of the bone depends on the magnitude of the load, and the orientation of the trabeculae depends on the orientation of the principle stresses that are applied.
So let me write that down. So they cut off the end of the bone, and they insert the implant into the hollow shaft of the remaining bone. And the metals they use are titanium, stainless steel, and a chromium-cobalt alloy. And then the bone grows into that implant.
And the bone grows in response to mechanical loads. So the density of the bone depends on the magnitude of the stresses, and the orientation of the bone depends on the principle stresses.
So one of the issues that comes up in joint replacements is that there's a mismatch in the moduli between the metal and the bone. So if you think the metal, like something like stainless steel, has a modulus of around 200, 210 gigapascals. And the cortical bone has a modulus of about 18 gigapascals, and the trabecular bone has a modulus between about 0.01 and 2 gigapascals, depending on its density. So you're taking the bone out, and you're replacing it with something that's much, much stiffer, and that changes the stress distribution around the remaining bone.
And one of the things that can happen is you can get a loosening of the implant. So the bone can grow in initially, but over time, you get a different stress field in the bone. And if you have a different stress field, then the bone can resorb away from the implant and cause what's called loosening. So if the implant becomes loose, that's clearly not a good thing. It's a bad thing.
And often orthopedic surgeons don't like to do these joint replacements in young people partly because they don't always loosen, but occasionally they do. And if they loosen they can go back and do a revision. But you can kind of imagine after they've chopped the head of the femur off and they put one implant in, it's not that easy to go back in and replace that with another one. You would need one with a longer stem, and the whole thing becomes a little bit more complicated.
So this issue of stress shielding is what it's called when you have something much stiffer that's shielding the stresses in the bone. The issue of stress shielding means that they don't like to do the replacements on younger patients unless you can get stress shielding.
And if we just compare-- if we look at the cobalt and chromium alloy, the modulus of that in gigapascals is about 210. If we look at the titanium alloys that are used, the modulus is about 110. If we look at the stainless steel-- it's 316 stainless steel-- it has a modulus of around 210. And then if we look at the bone, the cortical bone has a modulus of about 18, and the trabecular bone has a modulus 0.01 to 2 gigapascals depending on the density.
So after the joint replacement happens, the remodeling of the bone is affected. So the idea is that the stiffer metal carries more of the load, and then the bone carries less load, and then it resorbs. And that can lead to this thing called loosening, which is not desirable.
Now, this typically doesn't happen till about 15 years after you've had the implant, so it's not something that would happen right away, but it can happen later on. So these are all sort of medical reasons why people are interested in trabecular bone because of osteoporosis, osteoarthritis, and joint replacements.
So I wanted to start by talking about the structure of trabecular bone. And then we'll talk about what the stress-strain curves look like in compression and tension, what are the mechanisms of deformation and failure, and how we can apply our models for foams to the trabecular bone.
So the idea is that the structure of the bone resembles a foam, and here's some SCM images of trabecular bone. And you can see that the bone has a varying structure. If it's relatively low density, this is a bone that's almost like an open-cell foam if I didn't tell you that was a bone, you might actually think it was an open-cell foam.
And here's a denser piece of bone, and you can see there's still interconnections between all the openings, so it's not exactly like a closed-cell foam, but it's much denser, and it's almost like there's perforated plates in the structure. And then as I said the bone can grow in response to loads. So if you have loads that are more or less vertical, the trabeculae tend to line up and be more or less vertical with some sort of horizontal bracing.
So this is a piece of bone from a knee, the condyle is sort of towards the top of the knee. And you can see these are sort of plate-like pieces of bone. They're almost parallel, and not too surprisingly in your knee, the loads are typically vertical, and then there's a little bracing bits that go horizontally here. So you can get different structures depending on the loading on the bone, and the density of the bone corresponds to the magnitude of the load, and the orientation of the trabeculae corresponds to the orientation of the load.
AUDIENCE: [INAUDIBLE].
LORNA GIBSON: Resorb. So when the bone density goes down, when you lose bone mass, that's called resorption. So the idea is that the trabecular bone resembles a foam. And in fact, the word trabecular comes from Latin, and in Latin, it means little beam. So the foams to form by bending. They act like little beams, and so the trabeculae are like little beams, even in Latin.
There's a range of relative densities, and you can see in that image up there, you can see that there's a range. And they range typically between about a 5% in dense and 50% in dense. So something like 0.1 or 0.2 might be typical. And the low-density bone resembles an open-cell foam. And the higher density, it becomes more like perforated plates. And the structure can be highly anisotropic depending on the stress field.
And then I've got another image here of the trabecular bone. These images are using what's called micro computed tomography. So you've probably heard of computed tomography. Say somebody has cancer, they get put in a CT machine, and they do a scan. The micro CT is more of a research tool. It's the same kind of technology, but it's got a much finer resolution, and typically, you put a small specimen into a machine to do this.
So the specimen might be half an inch in diameter and an inch tall, something like that. So these are done by a colleague, Ralph Muller, who's in Zurich, and this is one of his bread and butter things that he has these images, and he looks at osteoporosis. And you can see here the difference in the structure for the different densities.
So here's a 26% dense piece of bone in the femoral head. It looks pretty sturdy and substantial. Here's an 11% dense piece from the lumbar spine, and here's a 6% dense piece. And you can kind of see when you go from 26 to 11, the struts get a little bit thinner. And when you go from 11 to 6, the struts get very thin, and in fact, if they get too thin, the struts resorb altogether, and some of their struts can just disappear.
So when people get osteoporosis, what happens is they first lose bone mass by thinning the struts, but then at some point, the struts just resorb altogether. And if you think of the struts as a biological material, they have bone cells in them. So there's little osteoclasts and osteoblasts and osteocytes that live in the bone, the mineral thing, the bony thing. And those cells have dimensions of 10s of microns, so maybe 20, 30 microns, something like that. So the struts can't get any thinner than that. If they get thinner than that, then the cells can't live, and the thing just disappears altogether.
And you can think of from a mechanical point of view, if you lose density by thinning the struts, you can use our sort of foam equations. And say the density went from 0.2 to 0.1, you could make some estimate of how the modulus and how the strength would vary depending on our foam models. But if you lose density by resorbing the struts, the struts just disappear altogether, then it's as if you had a steel scaffold or a steel structure of a building. And now you're starting to remove columns and remove beams.
Yes, I know. That's not good, not good. And so we'll talk a little bit more about that when we talk more about osteoporosis, and you can see the consequences of that. But this image here kind of gives you a little bit of a picture of what the bone structure looks like as it gets less dense.
So I want to talk a little bit more about the bone growing in response to load. Let me rub off the board.
So you're probably already a little bit familiar with this idea. So when astronauts go up into space, they often do exercises where they have a treadmill, and they've got springs, and they're pulling on the springs to try to exercise themselves. And the reason they do that is when they're in microgravity, if they were doing some kind of exercise, they would lose bone mass. And they will get back to Earth where we have Earth gravity, and they would have a problem.
So you see it in astronauts, in microgravity. The other place you see this just in everyday life is in professional tennis players. People have done like x-rays of the bones of professional tennis players, and obviously, they have one arm that they hit the ball with their racquet. The bones in that arm actually get bigger because they're loading that bone over and over again pretty much every day when they're playing tennis, and they're not loading the other arm. So their two arms are not symmetrical because of this loading from hitting the racquet over and over.
And the people in 3032 have already seen this, but I couldn't resist bringing up the Guinea fowl experiments again. So obviously, you can only do x-rays on human. You can't sacrifice the humans and look at their bones, but you can with Guinea fowl. And so people have done experiments where they run Guinea fowl on treadmills, and they have one set of Guinea fowl that they run on the treadmill that's horizontal. They have another set of Guinea fowl that they run on a treadmill that's inclined to 20 degrees, so one would think they might have more stress on their bones from that, and then they have a control group that they don't run on the treadmill at all.
And then what they do is they have a forced plate on the treadmill so as the Guinea fowl is running, they measure the maximum force in they're taking high-speed video. And then they measure the angle of the knee at that point at which the force is maximum. And they can see there's a change in the angle of the knee when they put them on the inclined treadmill, not too surprisingly.
And then these are juvenile Guinea fowl that haven't completely matured their bones. And then after about six weeks of this, they sacrifice the Guinea fowl, and they do scans on the bone, and they look at the orientation of the bone, and they measure what's called the orientation of the peak trabecular density, which is a way of characterizing the orientation of the bone.
And they find that the angle of the knee when the Guinea fowl are running changes by about 14 degrees. And it turns out the angle of the bone, the orientation of the bone also changes by about 14 degrees. So the bone has remodeled to match that change in the forces that are applied as the Guinea fowl are running on a treadmill. So this is all a demonstration just to show that bone grows in response to load.
So let me write down some of this stuff. So I will say astronauts-- did you see Michael Collins is going to come to the talk at MIT? When I was a kid in '60s, he was one of the Apollo astronauts. He was like one of the first NASA astronauts.
Anyway astronauts, so in microgravity, they would lose bone if they don't exercise. And tennis players, the bones get larger in the arm that they hold the racket with. And then I'll just write a little bit of notes about the Guinea fowl experiments. So this was done by-- it's in a paper, Ponzer et al 2006.
So they have one set of Guinea fowl that run on a level treadmill, they have another set that run on a inclined treadmill, and it's inclined at 20 degrees. And then they have a control group that doesn't run on the treadmill. And then they measure the angle at the knee at the moment of peak force on the treadmill. And after six weeks, they sacrificed the Guinea fowl, and they measured the orientation of the peak trabecular density.
And they find that the knee flexion angle changed by 13.7 degrees. And if you compared the inclined versus the level treadmill, and they found the orientation of the peak trabecular density, which they called OPDD, also changed by 13.6 degrees. So the idea is that the orientation of the trabeculae changed to match the orientation of the loading.
Then I have a little video here. Do you like video? So I have a colleague who's at Harvard who studies animal locomotion, and they didn't do this set of experiments, but they do do experiments on Guinea fowl running on treadmills, and thought you might find this amusing. So let me see if I can make this work.
[VIDEO PLAYBACK]
-Sometimes you walk into a lab and you just think this is what science is all about.
-I just put the Guinea fowl on the treadmill, and this is something that we commonly do.
-Welcome to the Concord Field Station, a defunct Nike missile base turned scientific menagerie. It's owned by Harvard, and biologist Andy Biewener is the director here. So think of it as--
-A research lab facility for doing comparative biomechanics and physiology of largely animal movement.
-And the birds are just the tip of the iceberg.
-So do you want to see the baby goat and the emu?
-Obviously.
-OK. We keep it because it's sort of like a mascot. There used to be a lizard colony. You can hear the African greys. Then the jerboas are housed in this room here. This is where we originally did our pigeon flight studies.
So usually the ones with claws and sharp teeth and aggressive behaviors, you want to watch out for them.
-As you might expect. But did you know that Guinea fowl--
-They're really lovely to work with.
-Sometimes. Or that--
-Rats are not very good on treadmills.
-Yes. That's what a rat treadmill looks like. And this?
-And this was historically a treadmill of note, the treadmill that they first taught kangaroos on and showed that kangaroos stored energy in their tendons enough that they don't actually increase their metabolic rate when they hop at faster speeds.
-These are the kind of discoveries made here with the use of high-speed video and x-ray machines and semi cooperative animals. But beyond the basic biology, Biewener says engineers are using this research to build better robots, and it can help improve medical treatment for people with movement disorders. Today the big excitement at the lab is happening here.
Ivo Ros is studying how heart rate changes when cockatiels fly at different speeds. So this is a way to look at how much energy it takes to fly, and that cord is measuring heart rate. But instead of the birds flying faster, the wind changes speed.
-I'm going to turn it on then.
-It's hard to fly fast, and it's hard to fly slow, Ros says. So the expectation is that the heart rate should be shaped like a U. But so far Ros is finding that it's a flat line. It's like the bird goes into a stress reaction when it takes off. Is that just because of the wind tunnel? What Ros wants to know is--
- --whether or not they need to be stressed to fly in the first place.
-That's something that Ros is looking into, but today is mostly about training.
-Keep going. Come on.
-Imagine you're a cockatiel. A wind tunnel is kind of a strange experience.
-A bird in a wind tunnel has to confront the fact that the world is not moving past, which defies its normal sensory cues.
-Which pretty well sums up the Concord Field Station generally. For Science Friday, I'm Flora Lichtman.
[END PLAYBACK]
And if read The New York Times, Flora Lichtman used to work for NPR and would make these Science Friday videos for them. But now she has a gig doing things for The New York Times, and she does science videos still. I don't know if you quite call them videos, but what they do is they have these little paper puppets, and the paper puppets are animated and re-enact different episodes in science. And it's kind of amazing how they do these little science videos. So if you Google Flora Lichtman, you'll see more Science Videos with all sorts of things.
I guess the other interesting anecdote is I went to the Concord Field Station once. And I had done a study on quills and animals that have quills because the quills have a foamy structure in the middle. So they're carrot, and they have sort of like carrot-like structures. And they have an outer shell that's dense, and then they have a foamy thing in the middle.
Anyway I did this paper on quills and how they work mechanically. And Technology reviewed a little article about it, and they said they wanted to take a picture of me with a hedgehog. The hedgehogs are little European-- they're like little small, cute things.
And I said, well, if you can find a hedgehog, I'm happy to have my photograph taken. And they had a hedgehog at the Concord Field Station. So we went out there, and we had these big leather gloves and took a picture.
And I don't if it was Andy or I don't know who it was, but I said one of the people there, what did you do with a hedgehog? And he said, well, we tried to do the treadmill study. But hedgehogs are like porcupines. When they get scared, they curl up into a little ball.
And so they said they would put the hedgehog down under the treadmill, and they would start it up, and it would make a noise, and it would get scared it. And it would just go into a little ball and kind of slide along to the end, and then it would kind of get flopped off. So that was the end of the hedgehog experiments.
But they did have wallabies there the day I went. So they have all sorts of animals that they put on to treadmills, birds that they fly, so it's kind of interesting to go there.
But the main idea here is that there was this set of experiments with Guinea fowl that showed just how precisely the orientation of the bone matches the orientation of the loads.
AUDIENCE: Was there a difference between the control groups?
LORNA GIBSON: Ah, so I have slides. I have slides. Hang on a sec. I got distracted by my video. Sorry. So here's the sort of schematic of Guinea fowl on treadmill. And where's the little doo-da here? So on the level, the knee flexion angle was whatever this is, 76.3, and here was the 62.6, so the difference is 13.7.
And then this kind of table here summarizes these results. So this is the maximum trabecular density, and this is the angle. And here we have the incline. Let's see, the control was the yellow, and the level was the blue, and they've got the values for that peak trabecular density orientation.
So they've got that for the level. And then they looked at the difference between the incline and the level in the knee angle. That's what this thing here is. And then between the level and the control, there wasn't really any difference in the knee angle because the control ones, they were just walking around.
So that's the sort of slide that has the actual data on it. All right. And then I showed you the video.
All right. So we need to do a couple more things before we get to the modeling. Let me get a drink. So if we want to use the models for foams to try to describe the trabecular bone, we need to know something about the properties of the solid. Remember we used the properties of the solid in the models.
So we want to get the properties of the solid in the trabeculae, and there's a couple of ways you can do this. To get the moduli, you can use an ultrasonic wave propagation method, and you can measure a modulus that way. And if they do that, they measure a modulus between about 15 and 18 gigapascals. Another way to do it is to take a piece of bone, do a compression test on it, measure the modulus. Before you do the test, you put it in the micro CT machine, and you get a picture of the structure, and then you use that as the input to a finite element analysis.
So the finite element analysis is a computer numerical analysis to do mechanical calculations. And if you know what the modulus of the structure is, you can back out what the modulus of the solid must have been from the fine element thing. And those sorts of experiments also showed that the modulus was around 18. And it turns out that moduli is about the same as cortical bone, and the properties of the solid trabeculae are very similar to the solid cortical bone.
So let me scoot over here. So if you use an ultrasonic wave propagation, people have measured a modulus for the solid in trabecular bone of 18 gigapascals, or you can do a finite element calculation based on micro CT data for the structure. And then you measure the overall modulus for the trabecular bone, and then you back out the modulus of the solid.
And people who've done that have gotten values of around 18 gigapascals too, and that's very similar to what the cortical bone is. And so we're going to use the following properties for the solid in the trabecular bone. We're going to say the density is 1,800 kilograms per cubic meter. The Young's modulus is 18 gigapascals.
The yield strength has different values in tension and compression. It's about 182 megapascals in compression. And it's about 115 megapascals in tension.
So those are the solid properties.
So then if we look at the compressive stress-strain curves, they have the shape shown on the screen there. And you can see how similar the stress-strain curves are for those for a foam. So there's the same three regimes that we see for the foam. There is a linear elastic regime over here, there's a stress plateau here, and there's some densification regime here. These are three curves for three different relative densities.
As the relative density goes up, the stiffness goes up, the plateau stress goes up, and the densification strain goes down. So is the same as the foams that we've looked at before.
And if we look at the mechanisms of deformation and failure, people have looked at this. These are on a whale vertebrae. So these are tests that are done in a micron CT machine, again, by Ralph Muller's group. And here the specimen is unloaded, and here's the same specimen loaded. So you can see this platen has come down a little bit. And if you look at this column here, this trabecular here, you can see it's bent out and bowed out more.
And people have found that usually the linear elastic behavior is controlled by bending of that trabeculae, and the plateau stress is usually controlled by some sort of buckling. But it's not elastic buckling. You don't recover it. If you take a piece of bone and you compress it, it's going to have a permanent deformation. So it's inelastic buckling.
And I think we have some more pictures. This is another example from whale bone from Ralph Muller's group. So here's the bone unloaded. Here it's loaded to 4% strain, here it's to 8%. And you can start seeing right in this area here if you compare with up there, it's starting to form. And if you go up here to 12% strain, you see that strut right there. That was this guy up here, and you can see that it's buckled right over.
So people have made measurements like this in observations, and you can actually see the buckling. And people have also done finite element modeling. They can take a micro CT scan and input that to the fine element model. And then if they do the compression and they input the properties of the solid, they can see that they get a buckling kind of failure.
If you have trabeculae that are very aligned-- we have more. Here's one more in the buckling. So this is one of Ralph's little movies. So when it unloads, it looks like it recovers, but this is all just an animation. He takes several stills and puts them together, and it doesn't actually recover. It's just the way that it shows.
But again, these are two different specimens of different densities. You can see how the struts deform. They bend and then they buckle.
Let me stop there, and I'll put some stuff on the board. So we'll say the compressive stress-strain curve has the characteristic shape of cellular solids. And the mechanisms of deformation and failure, usually there is bending followed by, usually, inelastic or plastic buckling.
And sometimes if the trabeculae are aligned like that knee that I showed you, or if the trabecular are aligned or if they're very dense, then the actual deformation is important. And I'll just say people have found this by making observations using micro computer tomography or by finite element calculations.
And this is a stress-strain curve and tension here, a tension you get failure at small strains, then you get micro cracks in the bone. And these next plots just show some data for the bone. So we're plotting the Young's modulus here. So this is a relative Young's modulus, the modulus of the bone divided by the solid cell wall material. Here's the relative density. Here's data for lots of different specimens of bone.
So some of this data is for human bones, some is for bovine bone. Sometimes the data is taken where the orientation of the trabeculae doesn't line up with the direction of the loading. So you might have trabeculae that are oriented this way, but you're loading it this way. There's sometimes different strain rates. Let's see. There's different groups, and so there's a huge scatter in the range of the data.
But you can see if you look at it broadly and you look at that whole cluster of data, the data lie close to a line of a slope of 2. And if you think of the open-celled foam model and you had bending of the cell walls, you'd expect that the modulus would vary [? to the ?] density squared. So that's kind of the limit of how we do the modeling. We're really just interested in seeing how the properties vary with density. If you had a particular piece of bone, I don't think you could use the models to exactly predict what the modulus of that bone would be.
And here's the compressive strength here. So this is the relative compressive strength. Here we've normalized it with the yield stress of the solid bone, and here's the relative density. And you can see, again, this line is of slope 2, so that kind of speaks to the buckling-type failure mode.
And I think I have another one here. This is the tensile strength. So if you pull the bone in tension, you wouldn't expect to get buckling, you'd expect to get plastic yielding. And if you got yielding and you use the open-cell foam model, you'd expect a slope of 3/2, so this line has a slope of 3/2. And this line is sort of towards the upper bound of that set of data. You can imagine a line that went through it a little bit lower but the same slope.
And so these open-celled foam models, they don't predict the properties of a particular piece of bone because the bone can have some anisotropy to it. The orientation of these things may not be perfectly lined up with the loading. But overall the models give you a sense of how the bone is deforming and failing.
So let me write some of this down. So that's data for the modulus, the compressive strength, and the tensile strength. And those have been on those plots. Those values are normalized by data for cortical bone. I thought somebody was talking. It's just the chair squeaking.
And as I said the spread in the data is large, and that's due to anisotropy in the bone and misalignment between the bone orientation and the loading direction. So when people first started doing tests on trabecular bone, they typically were orthopedics labs. And the orthopedics labs tended to initially cut the bone specimens on anatomical axes. So they would do you know the superior-inferior, or the medial-lateral, or the posterior-anterior. But the bone orientation didn't line up with those directions. So the bone might have been this way, but they were loading it this way, and so that gave this misalignment.
And there could be some variation in the solid properties too. So you could imagine some solid might have more micro cracks than another. So if you took say human bone of different ages, you might expect the older bone to have more micro cracks in it. So these plots put a lot of data together, and then the lines are based on models for open-cell foams.
So the relative modulus goes roughly as a relative density squared, and the cell walls are bending. And the compressive strength goes roughly as the modulus squared, and that's related to this plastic buckling. And then the tensile stress or tensile strength depends on the formation of plastic hinges, and it goes roughly as the density to the 3/2 power.
And one observation that people have made is that in compression, if the modulus and the strength both go as a density squared, then the ratio of the strength to the modulus is just a constant, and that, in fact, is just the strain at failure, or the strain for that say, the plateau. And that's a strain of about 0.7%, and that's pretty consistent in trabecular bone.
Let's see. And we said sometimes the bone was relatively aligned. So here's that picture of the femoral condyle again in the knee, and you can see the bones lined up. If you have bone that's lined up like that and you load it along the direction of alignment, then you can get axial deformation in the trabeculae. And then you would expect the moduli would go linearly with the density.
And here's some data for the Young's modulus and the compressive strength of bone that was fairly aligned. So this was selected to be aligned. So here's the modulus here. And the square data points are the longitudinal direction, and the little diamond, these little stars, are transverse. So here's a line of slope 1, and again, they don't all lie perfectly on that line, but roughly the slope is about 1 there.
And then similarly here, this is the compressive strength. Now the little squares are the longitudinal data, and they're not exactly on a slope of 1, but they're more or less on a slope of 1. So I'll just say in some regions, the bone may be aligned. And then axial deformation is important. And then you would expect the modulus to go linearly with the density and the strength to go linearly with the density in the longitudinal direction.
Then finally I wanted to finish up the bit on the modeling by making one of these plots a little bit like we did for wood. So here's the Young's modulus of bone plotted against the density. The trabecular bone is down here. It's sort the lowest density. And then this is the collagen that's in the solid part of the bone, and this is hydroxyapatite, the mineral. So the modulus of hydroxyapatite is around 120 gigapascals, and the modulus of collagen is somewhere around 5.
And if you make composites of collagen and hydroxyapatite, their moduli are going to be in this envelope here, and compact bone, the modulus fits in around here. Remember I said it was around 18 gigapascals. So then if you take a compact bone and you turn it into trabecular bone, you'd expect the modulus would go down along a slope of 2. So here's our little slope of 2, and more or less that's what you see with the trabecular bone.
So the idea is that the models give you kind of a general idea of how the bone is behaving, but it's not really meant to predict a particular piece of bone. Because a particular piece is going to have a particular geometry. Typically they're not equi ax and isotropic.
All right. So are we good with the general overview? Are we good with how fewer equations there are now that we got past the first part of the course? So I'm going to talk a bit more about osteoporosis, and I'm going to talk about some modeling that my group did to look at the consequences of osteoporosis. And then later on we're going to talk a little bit about using metal foams as a possible replacement material for a trabecular bone as well.
And I have a little bit of a talk on using trabecular bone in evolutionary studies to see whether or not a species was bipedal or quadrupedal. So I think I talked about this a little bit in 3032, but I have more slides and more stuff I'm going to talk about. So let me get myself organized.
So osteoporosis comes from the Latin, and it actually means porous bones. So osteo means bone, and not too surprisingly porosis means porous.
So this next slide gives you some idea what osteoporotic bone looks like. So the top slide is normal bone in a 55-year-old woman. These are sections from the lumbar spine. And that bone up here is 17% dense, so the relative density is point 0.17. And this is a section from the same area of bone in an 86-year-old woman, and it's 7% dense. So you can see there's a huge difference in the density, and you can start to see what happens when you lose bone mass.
So if you look at this bone up here, it's all well connected. Each little trabeculae is connected to its neighboring friends. And you can see down here, I mean, you look at this and you kind of go ouch just looking at it. Because this piece of bone here is just kind of dangling off, not connected to anything. And you can see the struts have gotten thinner, so they've lost bone mass by thinning. And then as I said when the thickness gets less than they are roughly equal to the size of the cells, then the cells can't live anymore, and the bone strut just disappears altogether.
So it's not too surprising that if you lose this much bone mass, there's mechanical consequences, and there's a greater risk of fracture. And as I said the two most common types of fractures are hip fractures and vertebral fractures.
So let's see here. So as people age, everybody loses bone mass. And happily for you and not so happily for me, the bone mass peaks at about 25 years old. So you're probably either not at the peak or just barely at the peak. And then it decreases after that every year. I'm considerably older than you.
And in women, when you go through menopause, the cessation of estrogen production increases the bone loss. And so typically, osteoporosis is most common in post menopausal women. And osteoporosis is defined as a bone mass 2.5 standard deviations or more below that of a young, normal mean. So it's not like you fall and break your hip and they say you have osteoporosis. It's based on the bone mass.
And as I said, the trabeculae thin and then they resorb completely. So anybody here take Latin? Yes. I did Latin for one year in high school. So trabeculae, with an E on the end here, trabeculae, I suppose, is the plural. Trabecula with an A is singular. And that comes from Latin. So you don't say trabeculas. That's a no-no.
All right. Let me get rid of these. So if we saw that the strength of the bone varies as the density squared, you can begin to see how sensitive the strength is going to be to this bone mass loss. So say you went from a density of 0.2 to a density of 0.1, then the densities changed by a factor of 2 so that the densities gone down by 1/2, but the strength is going to go down by a factor of 4. You're going to have the strength to be a 1/4. And so you're going to have a big change in the strength.
And you can imagine is the trabeculae thins, this buckling gets easier to happen. And then once the trabeculae begin to resorb as they disappear altogether, it's like I said. it's like having a building's framework. Now, you're removing beams and columns, and the strength is going to go down even more dramatically.
And the way we model the osteoporotic bone is we use finite element analysis. So before we talked about using the unit cell for the honeycomb. But to use the unit cell, you have to have repeating unit cells, and obviously, you don't have that. You've got local variations in what the structure looks like. And we also used a dimensional analysis. And the dimensional analysis relies on the geometry being similar from one specimen to another, and you can't really rely on that either for the osteoporotic bone.
And so what we've done is-- and this is what other people do as well, is use finite element modeling to represent the bone. So initially what we did was we used a 2D Voronoi model. So remember we talked about Voronoi honeycombs and Voronoi foams. So I like to start out with simple things, so we started out with 2D Voronoi model for a honeycomb.
Then we did a 2D representation of vertebral bone. And then we had a 3D Voronoi. And I had a couple of students who did this. Matt Silver was the one who did the first two, and Sereca Vagilla was the one who did the last one.
So let's see. I think that's probably a good place to stop there for today. So next time I'll talk about the modeling of osteoporotic bone, and we might talk a little bit about metal bones as a substitute for trabecular-- metal foams as a substitute. I don't think we'll get to the evolution stuff. We probably won't quite finish next time.
Free Downloads
Video
- iTunes U (MP4 - 167MB)
- Internet Archive (MP4 - 167MB)
Subtitle
- English - US (SRT)